β-Nicotinamide adenine dinucleotide: Biosynthesis, physiological function and application
General description
β-Nicotinamide adenine dinucleotide (NAD) is a cofactor central to metabolism. Found in all living cells, β-Nicotinamide adenine dinucleotide is called a dinucleotide because it consists of two nucleotides joined through their phosphate groups. One nucleotide contains an adenine nucleobase and the other nicotinamide. β-Nicotinamide adenine dinucleotide exists in two forms: an oxidized and reduced form, abbreviated as NAD and NADH (H for hydrogen) respectively. Its appearance is as follows:
Figure 1 Appearance of β-Nicotinamide adenine dinucleotide.
Characteristics
β-Nicotinamide adenine dinucleotide consists of two nucleosides joined by a pair of bridging phosphate groups. The nucleosides each contain a ribose ring, one with adenine attached to the first carbon atom (the 1' position) (adenosine diphosphate ribose) and the other with nicotinamide at this position. The nicotinamide moiety can be attached in two orientations to this anomeric carbon atom. Because of these two possible structures, the compound exists as two diastereomers. It is the β-nicotinamide diastereomer of β-Nicotinamide adenine dinucleotide that is found in organisms. These nucleotides are joined together by a bridge of two phosphate groups through the 5' carbons [1]. β-Nicotinamide adenine dinucleotide concentrations are highest in the mitochondria, constituting 40% to 70% of the total cellular NAD [2]. β-Nicotinamide adenine dinucleotide in the cytosol is carried into the mitochondrion by a specific membrane transport protein, since the coenzyme cannot diffuse across membranes. The intracellular half-life of NAD was claimed to be between 1-2 hours by one review, whereas another review gave varying estimates based on compartment: intracellular 1-4 hours, cytoplasmic 2 hours, and mitochondrial 4-6 hours [3].
Biosynthesis
β-Nicotinamide adenine dinucleotide is synthesized through two metabolic pathways. It is produced either in a de novo pathway from amino acids or in salvage pathways by recycling preformed components such as nicotinamide back to β-Nicotinamide adenine dinucleotide. Although most tissues synthesize β-Nicotinamide adenine dinucleotide by the salvage pathway in mammals, much more de novo synthesis occurs in the liver from tryptophan, and in the kidney and macrophages from nicotinic acid [4]. Most organisms synthesize β-Nicotinamide adenine dinucleotide from simple components. The specific set of reactions differs among organisms, but a common feature is the generation of quinolinic acid (QA) from an amino acid—either tryptophan (Trp) in animals and some bacteria, or aspartic acid (Asp) in some bacteria and plants. The quinolinic acid is converted to nicotinic acid mononucleotide (NaMN) by transfer of a phosphoribose moiety. An adenylate moiety is then transferred to form nicotinic acid adenine dinucleotide (NaAD). Finally, the nicotinic acid moiety in NaAD is amidated to a nicotinamide (Nam) moiety, forming β-Nicotinamide adenine dinucleotide.
Physiological function
β-Nicotinamide adenine dinucleotide has several essential roles in metabolism. It acts as a coenzyme in redox reactions, as a donor of ADP-ribose moieties in ADP-ribosylation reactions, as a precursor of the second messenger molecule cyclic ADP-ribose, as well as acting as a substrate for bacterial DNA ligases and a group of enzymes called sirtuins that use NAD+ to remove acetyl groups from proteins. In addition to these metabolic functions, NAD+ emerges as an adenine nucleotide that can be released from cells spontaneously and by regulated mechanisms, and can therefore have important extracellular roles [5].
Application
The enzymes that make and use NAD and NADH are important in both pharmacology and the research into future treatments for disease.Drug design and drug development exploits β-Nicotinamide adenine dinucleotide in three ways: as a direct target of drugs, by designing enzyme inhibitors or activators based on its structure that change the activity of NAD-dependent enzymes, and by trying to inhibit β-Nicotinamide adenine dinucleotide biosynthesis. Because cancer cells utilize increased glycolysis, and because β-Nicotinamide adenine dinucleotide enhances glycolysis, nicotinamide phosphoribosyltransferase (β-Nicotinamide adenine dinucleotide salvage pathway) is often amplified in cancer cells. It has been studied for its potential use in the therapy of neurodegenerative diseases such as Alzheimer's and Parkinson's disease [6]. A placebo-controlled clinical trial of NADH (which excluded NADH precursors) in people with Parkinson's failed to show any effect [7].
References
[1]Pollak et al. The power to reduce: pyridine nucleotides – small molecules with a multitude of functions. Biochem. J. 2007, 402 (2): 205–218.
[2]Hopp et al. Regulation of Glucose Metabolism by NAD + and ADP-Ribosylation. Cells. 2019, 8 (8): 890.
[3]Zhang et al. Regulatory Effects of NAD + Metabolic Pathways on Sirtuin Activity. Progress in Molecular Biology and Translational Science. 2018, 154: 71–104.
[4]McReynolds et al. Age-related NAD + decline. Experimental Gerontology. 2020, 134: 110888.
[5]Billington et al. Emerging functions of extracellular pyridine nucleotides. Mol. Med. 2006, 12 (11–12): 324–327.
[6]Belenky et al. NAD+ metabolism in health and disease. Trends Biochem. Sci. 2007, 32 (1): 12–9.
[7]Swerdlow et al. Is NADH effective in the treatment of Parkinson's disease?. Drugs Aging. 1998, 13 (4): 263–268.
You may like
Related articles And Qustion
Lastest Price from β-Nicotinamide adenine dinucleotide manufacturers

US $0.00-0.00/Box2025-05-07
- CAS:
- 53-84-9
- Min. Order:
- 1Box
- Purity:
- 99.99%
- Supply Ability:
- 3000box
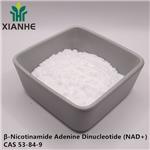
US $0.00/kg2025-04-30
- CAS:
- 53-84-9
- Min. Order:
- 1kg
- Purity:
- 0.99
- Supply Ability:
- 1000kg