How to synthesize D-Phenylalanine
Description
D-amino acids are intermediates for synthesising blactam antibiotics and other pharmaceuticals[1]. Chemical, fermentative, and enzymatic methods have already been developed to synthesize D-amino acids. Enzymatic methods are most suited for the industrial manufacture of D-amino acids regarding their optical purity and productivity and offer an efficient, highly specific and environmentally friendly alternative to chemical and fermentation methods. For the enzymatic methods, the hydantoinase-carbamoylase method is the primary method used for the commercial production of D-amino acids. In this two-step process, the DL-5-monosubstituted hydantoin used as a starting material is hydrolyzed by D-hydantoinase. The resulting N-carbamoyl-D-amino acid is hydrolyzed by carbamoylase to yield the free D-amino acid stereospecifically. However, the activity of the carbamoylase is much lower than that of D-hydantoinase, and the enzymes are relatively unstable, making the process unsuitable for industrial application.
Uses
D-Phenylalanine is an important chiral component of nateglinide, a drug for the treatment of type 2 diabetes, and widely used as intermediates in the synthesis of antibiotics, antiviral, analgesic and antistress agents. D-phenylalanine (D-Phe), a non-protein amino acid, is a building block for pharmaceuticals and fine chemicals.
Synthesis
Currently, D-Phe is produced mainly via enzymatic methods, including (i) hydantoinase processes, (ii) transamination, (iii) resolution of racemate, and (iv) reductive amination. The latter, which is catalyzed by meso-diaminopimelate dehydrogenase (DAPDH, EC 1.4.1.16), has the greatest potential as it can covert phenylpyruvic acid (PPA) and NH4Cl to D-Phe in a single step with high enantioselectivity[2].
Various enzymatic methods have been developed to synthesize D-Phe: (1) In the hydantoinase process, racemic 5-benzylhydantoin acts as a substrate for a cascade that involves hydantoin racemase, D-hydantoinase, and N-carbamoyl-D-amino acid amidohydrolase. D-Phe is produced at a high yield (45.9 g L-1, 98% molar yield) and 99% enantioselectivity in 48 h; however, the low solubility of substrate represents a rate-limiting step and leads to prolonged conversion times. (2) In transamination processes, PPA and D-glutamate act as co-substrates, with PPA being transaminated to D-Phe via D-transaminase, while D-glutamate is converted to α-ketoglutarate. During this catalytic process, NAD+ and formate are fed to the transformation broth to provide enough NADH. Glutamate dehydrogenase and glutamate racemase help recycle α-ketoglutarate back to D-glutamate, while FDH restores the reducing power (NADH). This process has yielded 58 g L-1 D-Phe with 100% ee in 35 h. (3) Asymmetric resolution processes utilize D/L-Phe as substrate and phenylalanine ammonia-lyase for the resolution from racemate. Although enantioselectivity can be quite high (>99%), the conversion rate of this process is limited to 50%, making downstream purification more difficult.
To this end, a tri-enzymatic cascade, which included PmLAAD, PvDAPDH, BmGDH, and L-Phe as substrate, was designed and reconstructed in vivo. As illustrated in the Figure shown above, D-Phe synthesis from L-phenylalanine (L-Phe) occurs in two steps: first, L-Phe undergoes oxidative deamination by L-amino acid deaminase (LAAD, EC 1.4.3.2) to produce the prochiral intermediate PPA; second, PPA undergoes reductive amination by DAPDH to generate D-Phe, with GDH (EC 1.1.1.47) coupling to regenerate NADPH. To reconstruct the cascade pathway in vitro, Proteus vulgaris PvDAPDH, P. mirabilis PmLAAD variant, and Bacillus megaterium BmGDH were selected based on their specific enzymatic activities. The three selected genes were amplified, overexpressed, and purified. To assess the feasibility of the proposed in vitro reconstruction system, the three enzymes were combined in an equimolar ratio and reacted with 5 g L-1 L-Phe, 0.5 mM NADP+, 1 g L-1 NH4Cl, and 7 g L-1 glucose for 4 h, after which 1.2 ± 0.1 g L-1 D-Phe was detected. The identity of the final product was confirmed using mass spectrometry and NMR analysis. This result demonstrated that the designed cascade composed of PvDAPDH, PmLAAD, and BmGDH successfully converted L-Phe to D-Phe. The effect of altering the BmGDH: PvDAPDH activity ratio (from 0.1:1 to 3:1) was investigated with 20 g L-1 PPA and DAPDH activity fixed at 4 U mL-1. When the BmGDH: PvDAPDH activity ratio reached 0.3:1, the D-Phe titer stabilized at 18.5 ± 0.4 g L-1, with 92.7% conversion rate. Similarly, when the PmLAAD: PvDAPDH activity ratio was set to 0.6–1.0:1, the D-Phe titer was 16.5 ± 0.4 g L-1, with 82.7% conversion rate. Therefore, the optimum PmLAAD:PvDAPDH: BmGDH activity ratio was set to 0.6–1.0:1:0.3.
References
[1] Longbao Zhu. “Efficient preparation of enantiopure D-phenylalanine through asymmetric resolution using immobilized phenylalanine ammonia-lyase from Rhodotorula glutinis JN-1 in a recirculating packed-bed reactor.” ACS Applied Bio Materials (2014): e108586.
[2] Cui Lu. “Efficient Synthesis of D-Phenylalanine from L-Phenylalanine via a Tri-Enzymatic Cascade Pathway.” ChemCatChem 13 13 (2021): 3165–3173.
You may like
Related articles And Qustion
Lastest Price from D-Phenylalanine manufacturers
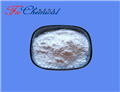
US $0.00/Kg/Drum2025-04-21
- CAS:
- 673-06-3
- Min. Order:
- 1KG
- Purity:
- 98%-101%
- Supply Ability:
- 500kgs
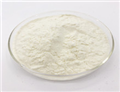
US $10.00/KG2025-04-21
- CAS:
- 673-06-3
- Min. Order:
- 1KG
- Purity:
- 99%
- Supply Ability:
- 100 mt