Glyoxal as an alternative fixative to formaldehyde
Abstract
Paraformaldehyde (PFA) is the most commonly used fixative for immunostaining of cells, but has been associated with various problems, ranging from loss of antigenicity to changes in morphology during fixation. We show here that the small dialdehyde glyoxal can successfully replace PFA. Despite being less toxic than PFA, and, as most aldehydes, likely usable as a fixative, glyoxal has not yet been systematically tried in modern fluorescence microscopy. Here, we tested and optimized glyoxal fixation and surprisingly found it to be more efficient than PFA‐based protocols. Glyoxal acted faster than PFA, cross‐linked proteins more effectively, and improved the preservation of cellular morphology. We validated glyoxal fixation in multiple laboratories against different PFA‐based protocols and confirmed that it enabled better immunostainings for a majority of the targets. Our data therefore support that glyoxal can be a valuable alternative to PFA for immunostaining.
Introduction
The 4% paraformaldehyde (PFA) solution has been a standard fixative for immunostaining and fluorescence microscopy, for several decades. Nevertheless, the literature contains numerous reports that PFA causes morphological changes, loss of epitopes, or mislocalization of target proteins and that it fixes the samples slowly and incompletely (see, e.g., Melan, 1994; Tanaka et al, 2010; Schnell et al, 2012). Many other fixatives have been introduced to alleviate these problems. Among them, glutaraldehyde is probably the most commonly used, since it fixes the samples faster and more completely than PFA (Smith & Reese, 1980). Mixtures of PFA and glutaraldehyde result in accurate fixation and reduce the lateral mobility of molecules (Tanaka et al, 2010), presumably by increasing the level of protein cross‐linking. However, this fixative mixture also reduces the efficiency of immunostainings, by blocking the antibody access to epitopes, or by causing particular epitopes to unfold (Farr & Nakane, 1981). Alcohol‐based fixation, such as treatments with ice‐cold methanol (Tanaka et al, 2010), results in stable fixation for a sub‐population of cellular structures (such as microtubules), but leads to poor morphology preservation and to a loss of membranes and cytosolic proteins. Overall, the improvements in fixation induced by glutaraldehyde or methanol do not compensate for their shortcomings, thus in most cases leaving PFA as the current fixative of choice.
A superior alternative to PFA is needed, especially since artifacts that were negligible in conventional microscopy are now rendered visible by the recent progress in super‐resolution microscopy (nanoscopy; Eggeling et al, 2015). To find a fixative that maintains high‐quality immunostainings while alleviating PFA problems, we have tested several compounds. We searched for commercially available molecules, which could be readily used by the imaging community. These included different combinations of PFA and glutaraldehyde, picric acid (Hopwood, 1985), and di‐imido‐esters (Woodruff & Rasmussen, 1979), which, however, were not better than PFA in immunostaining experiments. We have also investigated different aldehydes. We avoided highly toxic compounds such as acrolein, which would not be easy to use in biology laboratories, and we also avoided large aldehydes (more than 4–5 carbon atoms), whose fixative properties are expected to mimic those of glutaraldehyde. The small dialdehyde glyoxal fits these two criteria, since it has a low toxicity (as already noted in the 1940s, Wicks & Suntzeff, 1943) and contains only two carbon atoms. Glyoxal is used, at low concentrations, in glycation and metabolism studies (Boucher et al, 2015), which ensures that it is commercially available. It can be used as a fixative and has even been once described, in 1963, to provide better morphology preservation to formaldehyde (Sabatini et al, 1963). It is almost unknown in fluorescence experiments. We were able to find one publication, from 1975 (Swaab et al, 1975), in which glyoxal was used in immunofluorescence on brain samples, albeit followed by sample freezing, and by procedures that are not compatible with modern, high‐quality microscopy. We could also find a few publications on histological stains using glyoxal (e.g., Umlas & Tulecke, 2004; Paavilainen et al, 2010), which further encouraged us to test this compound.
Results
Glyoxal preserves the cellular morphology more accurately than PFA and fixes proteins and RNAs more strongly
To determine the optimal conditions of glyoxal fixation, we tested its action at different pH values (Appendix Table S1). We found that glyoxal requires an acidic pH, roughly between 4 and 5, despite one previous study that suggests that it may also fix samples at a neutral pH (Sabatini et al, 1963). In addition, we found that the morphology of the samples was much improved upon addition of a low‐to‐medium concentration of alcohol (ethanol, 10–20%), which may act as an accelerator in the fixation reactions. Removing the ethanol, or adjusting the pH above or below the 4–5 range, resulted in poor sample morphology (Appendix Table S1). pH values of 4 or 5 provided similar results for most of our experiments (results obtained at pH 5 are shown in all figures, unless noted otherwise) and provided better morphology preservation for cultured neurons than PFA. We tested PFA at various pH values (4, 5, and 7), with or without ethanol, at room temperature or at 37°C (Appendix Table S1), without finding a condition where the morphology of the PFA‐fixed samples consistently bettered that of glyoxal‐fixed samples.
We then proceeded to compare PFA and glyoxal fixation quantitatively. We first tested the speed with which these fixative solutions penetrate the cell membrane, by monitoring the fluorescence of propidium iodide, a fluorogenic probe that binds nucleic acids, and cannot enter living cells (Davey & Kell, 1996). Paraformaldehyde fixation allowed propidium iodide entry into cultured cells only after ~40 min, while glyoxal was substantially faster (Fig 1A). The same was observed using the membrane‐impermeant styryl dye FM 1‐43 (Betz et al, 1992): Glyoxal fixation enabled significant FM 1‐43 penetration within 1–2 min (Fig 1B). The difference in membrane penetration is probably due to the ethanol present in the glyoxal fixative, since the addition of ethanol to the PFA solution enhances its penetration into cells in a similar fashion (Appendix Fig S1), albeit it did not improve immunostainings with PFA (Appendix Fig S1; we would like to point out that low pH values, 4 and 5, also failed to improve PFA immunostainings, as shown in the same Appendix figure). In the same experiments, FM 1‐43 addition enabled us to visualize endocytotic events that took place during PFA fixation. Such events could be observed in every fixed cell (Fig 1B) and indicated that the cells were still active during PFA fixation, from the point of view of membrane trafficking. No such events could be detected during glyoxal fixation.
Figure 1.
Comparison of cell penetration by PFA and glyoxal
Speed of propidium iodide (PI) penetration into fibroblasts during 60 min of fixation with either 4% PFA or 3% glyoxal. N = 3 independent experiments. Glyoxal fixation enables PI to penetrate far more rapidly into the cells.
Speed of FM 1‐43 penetration in similar experiments. The arrowhead points to one example of ongoing endocytosis during PFA fixation. N = 3–4 independent experiments. The general pattern of FM 1‐43 entry was similar to that of propidium iodide. Only the first 10 min are shown, to enable an optimal observation of the kinetics of the first stages of FM 1‐43 entry. The results parallel those obtained with PI: faster penetration during glyoxal fixation.
);You may like
Related articles And Qustion
See also
Lastest Price from Glyoxal manufacturers

US $15.00/kg2024-04-27
- CAS:
- 107-22-2
- Min. Order:
- 1kg
- Purity:
- 99.912%
- Supply Ability:
- 10ton
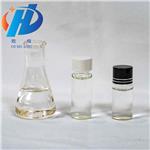
US $3.00/kg2024-04-22
- CAS:
- 107-22-2
- Min. Order:
- 1kg
- Purity:
- 99.92%
- Supply Ability:
- 50000tons