What is the Cyclic AMP Pathway?
Introduction
The cyclic nucleotide, cyclic 3’,5’-adenosine monophosphate (cyclic AMP), is the prototypical member of the family of second messengers, which mediates the intracellular actions of a wide range of cell surface receptors for hormones, neurotransmitters, growth factors, as well as sensory stimuli. Cyclic AMP is now recognized as a universal regulator of cellular function in a wide variety of organisms, including protozoa, plants, and animals. Cyclic AMP signaling is now known to occur in discrete sub-cellular compartments and is now widely viewed as the paradigm for “compartmentalized” cellular signaling in biological systems. Biological processes mediated by cyclic AMP include memory formation, metabolism, gene regulation, and immune function, and its deregulation is associated with many pathologies, including metabolic, cardiovascular, pulmonary, and inflammatory disorders, as well as certain cancers[1].
Biological activity
Cyclic AMP is synthesized intracellularly following activation of GPCRs by physiological stimuli, thereby translating extracellular ligand binding into intracellular signals by conformational changes in the receptor that traverse the cell membrane. These structural effects result in the dissociation of Ga and Gβγ heterotrimeric G-protein subunits in the plasma membrane 1. It is the stimulatory, the Gsa subunit, that is specifically involved in the synthesis of cyclic AMP since it activates adenylate cyclase (AC) at the plasma membrane, thereby catalyzing the conversion of ATP into cyclic AMP and pyrophosphate. In contrast, inhibitory Gia subunits block AC activation, limiting cyclic AMP production. Elevated intracellular cyclic AMP levels can activate a select range of signalling pathways through specific interactions with effector proteins that contain cyclic nucleotide binding domains (CNBs). These include protein kinase A (PKA), exchange protein activated by cyclic AMP (EPACs 1 and 2), cyclic AMP responsive ion channels (CICs), and Popeye domain-containing proteins (POPDCs). Activating these proteins by cyclic AMP controls many aspects of cellular function, including proliferation, differentiation, secretion, cell spreading, inflammation, contractility, and plasma membrane remodeling. As such, cyclic AMP signaling has become an attractive target for drug development for the treatment of a large variety of diseases.
Cyclic AMP Pathway
cAMP, as an essential messenger involved in regulating metabolism and biological functions in cells, transfers information regarding cellular status. With functions including the regulation of neurotransmitter synthesis, regulation of membrane protein activity, participation in ganglion synaptic transmission, and regulation of transcription factors in eukaryotic cells, cAMP may be involved in the prevention and treatment of various diseases. Therefore, detecting the level of cAMP is essential in investigating medically relevant signal transduction pathways.
Figure. The cAMP/PKA pathway.
There are three main effectors of cAMP: PKA, the guanine-nucleotide-exchange factor (GEF) EPAC, and cyclic-nucleotide-gated ion channels[2]. Protein kinase (PKA), the best-understood target, is a symmetrical complex of two regulatory (R) subunits and two catalytic (C) subunits (there are several isoforms of both subunits). It is activated by the binding of cAMP to two sites on each of the R subunits, which causes their dissociation from the C subunits. The catalytic activity of the C subunit is decreased by a protein kinase inhibitor (PKI), which can also act as a chaperone and promote nuclear export of the C subunit, thereby decreasing the nuclear functions of PKA. PKA-anchoring proteins (AKAPs) provide specificity in cAMP signal transduction by placing PKA close to specific effectors and substrates. They can also target it to particular subcellular locations and anchor it to ACs (for immediate local activation of PKA) or PDEs (to create local negative feedback loops for signal termination).
A large number of cytosolic and nuclear proteins have been identified as substrates for PKA. PKA phosphorylates numerous metabolic enzymes, including glycogen synthase and phosphorylase kinase, which inhibit glycogen synthesis and promote glycogen breakdown, respectively, and acetyl CoA carboxylase, which inhibits lipid synthesis. PKA also regulates other signaling pathways. For example, it phosphorylates and thereby inactivates phospholipase C (PLC) β2. In contrast, it activates MAP kinases; in this case, PKA promotes phosphorylation and dissociation of an inhibitory tyrosine phosphatase (PTP). PKA also decreases the activities of Raf and Rho and modulates ion channel permeability. In addition, it regulates the expression and activity of various ACs and PDEs.
Regulation of transcription by PKA is mainly achieved by direct phosphorylation of the transcription factors cAMP-response element-binding protein (CREB), cAMP-responsive modulator (CREM), and ATF1. Phosphorylation is a crucial event because it allows these proteins to interact with the transcriptional coactivators CREB-binding protein (CBP) and p300 when bound to cAMP-response elements (CREs) in target genes. The CREM gene also encodes the potent repressor ICER, which negatively feeds back on cAMP-induced transcription. However, the picture is more complex because CREB, CREM, and ATF1 can all be phosphorylated by many different kinases. PKA can also influence the activity of other transcription factors, including some nuclear receptors.
In addition to being negatively regulated by signals that inhibit AC or stimulate PDE activity, the action of PKA is counterbalanced by specific protein phosphatases, including PP1 and PP2A. PKA, in turn, can negatively regulate phosphatase activity by phosphorylating and activating specific PP1 inhibitors, such as I1 and DARPP32. PKA-promoted phosphorylation can also increase the activity of PP2A as part of a negative feedback mechanism.
Another critical effector for cAMP is EPAC, a GEF that promotes the activation of certain small GTPases (e.g., Rap1). A major function of Rap1 is to increase cell adhesion via integrin receptors (how this occurs is unclear).
Finally, cAMP can bind to and modulate the function of a family of cyclic nucleotide-gated ion channels. These are relatively nonselective cation channels that conduct calcium. Calcium stimulates CaM and CaM-dependent kinases and, in turn, modulates cAMP production by regulating the activity of ACs and PDEs. The channels are also permeable to sodium and potassium, which can alter the membrane potential in electrically active cells.
[1] Cells | Special Issue : New Advances in Cyclic AMP Signalling (mdpi.com) https://www.mdpi.com/journal/cells/special_issues/cyclic_AMP
[2] Sassone-Corsi, Paolo. “The cyclic AMP pathway.” Cold Spring Harbor perspectives in biology (2012).
You may like
Related articles And Qustion
See also
Lastest Price from Cyclic AMP manufacturers
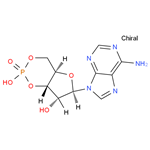
US $0.00/kg2024-12-17
- CAS:
- 60-92-4
- Min. Order:
- 1kg
- Purity:
- 90.0%Min. HPLC
- Supply Ability:
- 1000kg
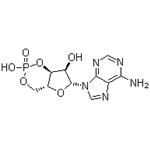
US $1500.00/kg2024-12-16
- CAS:
- 60-92-4
- Min. Order:
- 1kg
- Purity:
- 99.0%
- Supply Ability:
- 10tons