Novel synthesis of Ethylene glycol: Nexus molecule outputs pure ethylene glycol
As a cornerstone of industrial chemicals, ethylene glycol serves multiple functions. Not only does it find extensive application as an antifreeze agent, but it is also a critical precursor in the production of a diverse range of products such as polyester fibres, films and resins.
The currently dominant approach to ethylene glycol synthesis is grounded in a two-step energy-intensive process (Fig. 1a) that incorporates the generation of ethylene oxide as an integral intermediate step. However, this approach suffers from limited product selectivity owing to the overoxidation of ethylene. This, in turn, culminates in considerable carbon dioxide emissions. Progress in renewable energy has sparked evolutionary advances in electrified chemical manufacturing, establishing a promising route to sustainable production of ethylene glycol under ambient conditions.
Illustratively, the electrochemical oxidation of ethylene to
ethylene glycol has been successfully realized through the use of a
Pd-based electrocatalyst in an aqueous electrolyte, demonstrating
an alternative for ethylene glycol production at ambient temperature
and pressure. Nevertheless, the direct electrochemical oxidation of
ethylene encounters formidable hurdles, including a limited current
density (~5 mA cm−2), by-product formation (formic acid, acetaldehyde, glycolaldehyde), high catalyst cost, and overoxidation to form
CO2 at high overpotentials (Fig. 1b).
Now, writing in Nature Catalysis , Lei Wang and colleagues
describe a cascade catalytic approach that demonstrates remarkable
selectivity (~100%) and productivity in the conversion of CO2-derived
C2H4 to ethylene glycol. This strategy capitalizes on the use of electrochemically generated pure H2O2 as a nexus oxidant (Fig. 1c). In this
work, the cascade ethylene glycol production was initially demonstrated in an H-type reactor, in which titanium silicalite-1 (TS-1) particles were simply dispersed in the cathode chamber with a continuous
supply of O2 and ethylene. By using carboxyl-functionalized carbon
nanotubes as the electrocatalyst for the 2e−
oxygen reduction reaction,
H2O2 was produced as the desired oxidant, resulting in a maximum
ethylene glycol production rate of 0.23 mmol h−1. Furthermore, they
uncovered the pivotal role of the acidic electrolyte in the cascade
ethylene glycol production process. Specifically, although the electrochemical H2O2 production rates remained similar across all three types
of electrolytes (0.1 M H2SO4, 0.1 M K2SO4 and 0.1 M KOH), significant
ethylene glycol production was observed exclusively in the acidic
electrolyte. It was revealed that the high proton concentration in the
acidic electrolyte aids the transformation of the ethylene oxidation
intermediate into ethylene glycol. Additionally, the absence of alkali
ions in the acidic electrolyte effectively prevents catalyst poisoning
in TS-1 (ref. 7).
Wang et al. then conducted a methodical inquiry into the
kinetics associated with the production and consumption of H2O2.
Interestingly, despite the consistently high Faradaic efficiency of H2O2
— which reaches approximately 90% across the entire range of current
densities investigated — there is an observed reduction in the overall
electron-to-ethylene-glycol efficiency (ETE) as the total current density
increases. Through a series of control experiments, they concluded
that the decrease in ETE resulted from two factors: electrochemical
over-reduction of H2O2 to H2O and the self-decomposition of H2O2
catalysed by TS-1. To overcome these challenges, the authors proposed
a modified system that incorporates a solid-acid electrolyte. This
innovative approach not only tackles the constrained mass transport
in the H-type reactor, but also addresses the onerous and costly tasks
of downstream product separation and purification from the H2SO4
electrolyte. Initially, when directly integrating an H2O2 production
solid-electrolyte reactor with an ethylene oxidation reactor, it was
observed that the output consisted primarily of ethylene oxide, rather
than ethylene glycol. To solve this issue, a highly acidic solid electrolyte,
serving a similar function to H2SO4, was included as a co-catalyst to
enhance the efficiency of ethylene glycol production on the ethylene
oxidation reactor. Under optimized conditions, this cascade reactor achieved a rate of 2.4 mmol h−1 for ethylene glycol production
with an overall electronic efficiency of approximately 70%. By using
amine-functionalized carbon nanotubes as the electrocatalyst, the
production rate of ethylene glycol was further improved to around
5.3 mmol h−1. The authors further demonstrated the capability of generating highly concentrated ethylene glycol solution (up to approximately 0.5 M) using a 4 cm2
electrolyser. They have engineered a highly
advanced three-compartment electrolyser that excels at ethylene
oxidation, functioning effectively at both the anode and cathode.
The key innovation lies in the replacement of the traditional anodic water oxidation reaction with a chloride oxidation reaction. This configuration leads to the generation of hypochlorous acid in the anode, a highly effective oxidizing agent that plays a crucial role in converting ethylene into ethylene oxide.
Finally, the researchers successfully demonstrated the production of ethylene glycol from CO2 via a cascade catalytic system that
seamlessly integrates electrocatalysis and thermocatalysis. Based
on this, they conducted a preliminary techno-economic analysis
and underscored the economic viability of the proposed ethylene
glycol production process, with an estimated production cost
of US$1,031 t−1, compared with the current market price of about
US$1,400 t−1. The two primary cost components are electricity and
separation, so this outcome becomes particularly favourable when
the process uses renewable electricity sources at their currently low
but attainable prices. Additionally, achieving products with high concentration is desirable as the separation cost is closely tied to the concentration of the final product.
References:
[1] XIA C. Nexus molecule outputs pure ethylene glycol[J]. Nature Catalysis, 2023. DOI:10.1038/s41929-023-00989-2.
You may like
Related articles And Qustion
Lastest Price from Ethylene glycol manufacturers
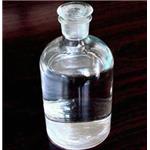
US $3.60/kg2025-04-18
- CAS:
- 107-21-1
- Min. Order:
- 1kg
- Purity:
- ≥99%
- Supply Ability:
- 3000tons/month
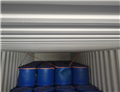
US $1.00/kg2025-04-18
- CAS:
- 107-21-1
- Min. Order:
- 1kg
- Purity:
- 99%
- Supply Ability:
- 10 mt