
CHLOROQUINE
- Product NameCHLOROQUINE
- CAS54-05-7
- MFC18H26ClN3
- MW319.87
- EINECS200-191-2
- MOL File54-05-7.mol
Chemical Properties
Melting point | 87° |
Boiling point | 475.41°C (rough estimate) |
Density | 1.0500 (rough estimate) |
refractive index | 1.6010 (estimate) |
storage temp. | 2-8°C(protect from light) |
solubility | Chloroform (Slightly), Methanol (Slightly) |
form | Solid |
pka | pKa 8.4(H2O t = 20) (Uncertain) |
color | White to Light Brown |
Odor | Odorless |
Stability | Stable, but light sensitive. Incompatible with strong oxidizing agents. |
IARC | 3 (Vol. 13, Sup 7) 1987 |
EPA Substance Registry System | 1,4-Pentanediamine, N4-(7-chloro-4-quinolinyl)-N1,N1-diethyl- (54-05-7) |
Safety Information
Hazardous Substances Data | 54-05-7(Hazardous Substances Data) |
Toxicity | LD50 oral in rat: 330mg/kg |
Usage And Synthesis
Chloroquine was first synthesized by Andersag in 1934 and the initial clinical studies were performed in Germany. The drug later underwent extensive clinical studies by the Americans in 1944 and was found to be an outstanding antimalarial compound. Chloroquine is a racemate with two enantiomers with similar antimalarial activity. It is a potent schizontocidal drug which is highly effective against the asexual forms of all four species of malaria. It is also active against gametocytes of Plasmodium (P.) vivax, P. malariae and P. ovale, but not against P. falciparum [1].
Development of chloroquine-resistant P. falciparum parasites is now widespread. Much work has been done to understand the mechanisms of this resistance. It is now well documented that red blood cells infected with resistant plasmodia concentrate less Chloroquine than those infected with susceptible strains[2,3]. This is due to an increased efflux of Chloroquine from chloroquine-resistant parasites [4]. It has also been demonstrated that the calcium channel blocker verapamil and a number of other drugs can inhibit this efflux and reverse Chloroquine resistance in vitro [5,6], but the doses of these inhibitors used in vitro are too high to be clinically useful. Its metabolite deethylchloroquine is less active in vitro against resistant P. falciparum strains, but not against susceptible strains [7].
The mechanism of action of Chloroquine is probably related to its inhibition of the enzyme that polymerizes and detoxifies ferriprotoporphyrin IX in the parasite food vacuole [8]. Chloroquine has also amoebicidal and anti-inflammatory properties [9].
Development of chloroquine-resistant P. falciparum parasites is now widespread. Much work has been done to understand the mechanisms of this resistance. It is now well documented that red blood cells infected with resistant plasmodia concentrate less Chloroquine than those infected with susceptible strains[2,3]. This is due to an increased efflux of Chloroquine from chloroquine-resistant parasites [4]. It has also been demonstrated that the calcium channel blocker verapamil and a number of other drugs can inhibit this efflux and reverse Chloroquine resistance in vitro [5,6], but the doses of these inhibitors used in vitro are too high to be clinically useful. Its metabolite deethylchloroquine is less active in vitro against resistant P. falciparum strains, but not against susceptible strains [7].
The mechanism of action of Chloroquine is probably related to its inhibition of the enzyme that polymerizes and detoxifies ferriprotoporphyrin IX in the parasite food vacuole [8]. Chloroquine has also amoebicidal and anti-inflammatory properties [9].
Despite the presence of resistant P. falciparum parasites, chloroquine is still with few exceptions, the drug of choice for treatment of non-severe malaria in the semi-immune indigenous population in Africa south of the Sahara. It should, however, not be used in severe or complicated malaria. In areas with considerable risk for chloroquine resistant P. falciparum, the drug has to be combined with another antimalarial drug, e.g. proguanil, for effective prophylaxis. Chloroquine still remains the drug of choice for treatment and prophylaxis of malaria caused by the other forms of plasmodia except in New Guinea.
Chloroquine is also useful for the treatment of amoebic hepatitis and in rheumatoid arthritis.
Chloroquine is also useful for the treatment of amoebic hepatitis and in rheumatoid arthritis.
The dosage used during standard treatment and prophylaxis of malaria is usually well tolerated. Minor side effects such as nausea, vomiting, diarrhoea, dizziness, headache, rash and abdominal pain may occur. Accommodation problems at the time of maximal concentration a few hours after intake can be disturbing but disappear rapidly. Many of the mild concentration dependent side effects can be avoided if chloroquine is taken with some food at bedtime [9]. Itching is common in blacks [10-12] and has been reported in Caucasians as well [13]. It is a subjective reaction characterized by a widespread pricking sensation affecting mainly the hands, feet and the scalp without any rash. It begins 6–12 hours after drug intake and usually subsides within 3–4 days after stopping treatment. Chloroquine has been reported to exacerbate psoriasis [14]. Acute cardiovascular effects have been reported to be associated with parenteral administration of chloroquine, [15] but can be avoided by slow intravenous infusion[16].
Chloroquine is known to carry a risk for toxic retinopathy when used in high doses for treatment of rheumatoid arthritis, particularly if the cumulative dose is above 100 g[17]. In a recent study of 588 missionaries who have taken a median cumulative dose of chloroquine of more than 300 g for malaria prophylaxis (some of them for up to 24 years), none of the subjects were reported to have retinopathy [18].
Rare side effects occasionally reported include photosensitivity, tinnitus, reduced hearing and deafness, neuromyopathy, involuntary movements and aplastic anaemia, agranulocytosis, thrombocytopenia and neutropenia [17].
Chloroquine is a well established but rare cause of toxic psychosis [19-21]. The symptoms disappear within two weeks of drug discontinuation. An association between epileptic seizures and chloroquine use has been reported[22]. During long-term treatment (e.g. of rheumatoid arthritis) with high doses of chloroquine side effects are more common. Most of them are related to the central nervous system and include diplopia, blurred vision, accommodation problems, apathy, anxiety, fatigue, headache, dizziness and vertigo. These side effects are probably concentration dependent .
Chloroquine is known to carry a risk for toxic retinopathy when used in high doses for treatment of rheumatoid arthritis, particularly if the cumulative dose is above 100 g[17]. In a recent study of 588 missionaries who have taken a median cumulative dose of chloroquine of more than 300 g for malaria prophylaxis (some of them for up to 24 years), none of the subjects were reported to have retinopathy [18].
Rare side effects occasionally reported include photosensitivity, tinnitus, reduced hearing and deafness, neuromyopathy, involuntary movements and aplastic anaemia, agranulocytosis, thrombocytopenia and neutropenia [17].
Chloroquine is a well established but rare cause of toxic psychosis [19-21]. The symptoms disappear within two weeks of drug discontinuation. An association between epileptic seizures and chloroquine use has been reported[22]. During long-term treatment (e.g. of rheumatoid arthritis) with high doses of chloroquine side effects are more common. Most of them are related to the central nervous system and include diplopia, blurred vision, accommodation problems, apathy, anxiety, fatigue, headache, dizziness and vertigo. These side effects are probably concentration dependent .
Chloroquine should not be given to persons with a history of epilepsy or psychosis. It should also be avoided in psoriatic patients. Since chloroquine is mainly excreted unchanged by the kidney, dosage reductions may be considered in patients with kidney impairment. Cardiovascular collapse may occur after parenteral administration of chloroquine in severely ill patients especially in children. The drug, must therefore be given as a slow intravenous infusion with adequate fluid replacement (see below).
Concurrent chloroquine intake may increase the mouth ulcerations reported with proguanil[23]. Cimetidine inhibits the metabolism of chloroquine and may cause increased plasma levels of the latter [24].
Numerous preparations (tablets, oral solutions, solutions for injection) containing chloroquine phosphate or sulphate are available. 161 mg chloroquine phosphate equals 100 mg chloroquine base. 136 mg chloroquine sulphate equals 100 mg chloroquine base.
1. Black RH, Canfield CJ, Clyde DF, Peters W, Wernsdorfer WH (1986). Quinine. In: Chemotherapy of Malaria 2nd edn, edited by L Bruce-Chwatt. Monograph series no. 27. (Geneva: World Health Organization).
2 Fitch CD (1969). Chloroquine resistance in malaria. A deficiency of chloroquine binding. Proceedings of the National Academy of Science of the USA, 64, 1181–1187.
3. Verdier F, Le Bras J, Clavier F, Hatin I, Blayo MC (1985). Chloroquine uptake by Plasmodium falciparum-infected human erythrocytes during in vitro culture and its relationship to Chloroquine resistance. Antimicrob Agents Chemother, 27, 561–564.
4. Krogstad DJ, Gluzman IY, Kyle DE, Odoula AMJ, Martin SK, Milhous WK, Schlesinger PH (1987). Efflux of chloroquine from Plasmodium falciparum: mechanism of Chloroquine resistance. Science, 238, 1283–1285.
5. Martin SK, Oduola AMJ, Milhous WK (1987). Reversal of chloroquine resistance in Plasmodium falciparum by verapamil. Science, 235, 899–901.
6. Bitonti AJ, Sjoerdsma A, McCann PP, Kyle DE, Oduola AMJ, Rossan RN, Milhous WK, Davidsson DE (1988). Reversal of chloroquine resistance in malaria parasite Plasmodium falciparum by desipramine. Science, 242, 1301–1303.
7. Aderounmu AF, Fleckenstein L (1983). Pharmacokinetics of chloroquine diphosphate in the dog. J Pharmacol Exp Ther, 226, 633–639.
8. Slater AFG, Cerami A (1992). Inhibition by chloroquine of a novel haem polymerase enzyme activity in malaria trophozoites. Nature, 355, 167–169
9. Martindale: The Extra Pharmacopoeia, 30th edn, (1993). Chloroquine and other antimalarials (London: Pharmaceutical Press), pp. 394–397.
10. Olatunde A (1977). The practical and therapeutic implications of chloroquine-induced itching in tropical Africa. Afr J Med Sci, 6, 27–31.
11. Ajayi AA, Oluokun A, Sofowora O, Akinleye A, Ajayi AT (1989). Epidemiology of antimalarialinduced pruritus in Africans. Eur J Clin Pharmacol, 37, 539–540.
12. Sowunmi A, Walker O, Salako LA (1989). Pruritus and antimalarial drugs in Africans. Lancet, 2, 213.
13. Poulter NR, Lury JD, Poulter CJ (1982). Chloroquine-associated pruritus in a European. BMJ, 285, 1703–1704.
14. Schopf RE, Ockenfels HM, Schultewolter T, Morsches B (1983). Chloroquine stimulates the mitogen-driven lymphocyte proliferation in patients with psoriasis. Dermatology, 187, 100–103.
15. Looareesuwan S, White NJ, Chanthavanich P, Edwards G, Nicholl DD, Bunch C, Warrell DA (1986). Cardiovascular toxicity and distribution kinetics of intravenous chloroquine. Br J Clin Pharmacol, 22, 31–36.
16. White NJ, Watt G, Bergqvist Y, Njelesani EK (1987). Parenteral chloroquine for treating falciparum malaria. J Infect Dis, 155, 192–201.
17. Tester-Dalderup CBM (1992). Antiprotozoal drugs: Antimalarial drugs. Meyler’s Side Effects of Drugs, 12th edn, pp 688–692.
18. Lange WR, Frankenfield DL, Moriarty-Sheehan M, Contoreggi CS, Frame JD et al. (1994). No evidence for chloroquine-associated retinopathy among missionaries on long-term malaria prophylaxis. Am J Trop Med Hyg, 51, 389–392.
19. Brokes DB (1966). Chloroquine psychosis. BMJ, I, 983.
20. Dornhorst AC, Robinson BF (1963). Chloroquine psychosis Lancet, i, 108.
21. Good MI, Shader RI (1977). Behavioral toxicity and equivocal suicide associated with chloroquine and its derivatives. Am J Psychiatry, 134, 798–801.
22. Fish DR, Espir MLE (1988). Convulsions associated with prophylactic antimalarial drugs: implications for people with epilepsy. BMJ, 297, 526–527.
23. Drysdale SF, Phillips-Howard PA, Behrens RH (1990). Proguanil, Chloroquine, and mouth ulcers. Lancet, 335, 164.
24. Ette EI, Brown-Awala EA, Essien EE (1987). Chloroquine elimination in humans: effect of lowdose cimetidine. J Clin Pharmacol, 27, 813–881.
2 Fitch CD (1969). Chloroquine resistance in malaria. A deficiency of chloroquine binding. Proceedings of the National Academy of Science of the USA, 64, 1181–1187.
3. Verdier F, Le Bras J, Clavier F, Hatin I, Blayo MC (1985). Chloroquine uptake by Plasmodium falciparum-infected human erythrocytes during in vitro culture and its relationship to Chloroquine resistance. Antimicrob Agents Chemother, 27, 561–564.
4. Krogstad DJ, Gluzman IY, Kyle DE, Odoula AMJ, Martin SK, Milhous WK, Schlesinger PH (1987). Efflux of chloroquine from Plasmodium falciparum: mechanism of Chloroquine resistance. Science, 238, 1283–1285.
5. Martin SK, Oduola AMJ, Milhous WK (1987). Reversal of chloroquine resistance in Plasmodium falciparum by verapamil. Science, 235, 899–901.
6. Bitonti AJ, Sjoerdsma A, McCann PP, Kyle DE, Oduola AMJ, Rossan RN, Milhous WK, Davidsson DE (1988). Reversal of chloroquine resistance in malaria parasite Plasmodium falciparum by desipramine. Science, 242, 1301–1303.
7. Aderounmu AF, Fleckenstein L (1983). Pharmacokinetics of chloroquine diphosphate in the dog. J Pharmacol Exp Ther, 226, 633–639.
8. Slater AFG, Cerami A (1992). Inhibition by chloroquine of a novel haem polymerase enzyme activity in malaria trophozoites. Nature, 355, 167–169
9. Martindale: The Extra Pharmacopoeia, 30th edn, (1993). Chloroquine and other antimalarials (London: Pharmaceutical Press), pp. 394–397.
10. Olatunde A (1977). The practical and therapeutic implications of chloroquine-induced itching in tropical Africa. Afr J Med Sci, 6, 27–31.
11. Ajayi AA, Oluokun A, Sofowora O, Akinleye A, Ajayi AT (1989). Epidemiology of antimalarialinduced pruritus in Africans. Eur J Clin Pharmacol, 37, 539–540.
12. Sowunmi A, Walker O, Salako LA (1989). Pruritus and antimalarial drugs in Africans. Lancet, 2, 213.
13. Poulter NR, Lury JD, Poulter CJ (1982). Chloroquine-associated pruritus in a European. BMJ, 285, 1703–1704.
14. Schopf RE, Ockenfels HM, Schultewolter T, Morsches B (1983). Chloroquine stimulates the mitogen-driven lymphocyte proliferation in patients with psoriasis. Dermatology, 187, 100–103.
15. Looareesuwan S, White NJ, Chanthavanich P, Edwards G, Nicholl DD, Bunch C, Warrell DA (1986). Cardiovascular toxicity and distribution kinetics of intravenous chloroquine. Br J Clin Pharmacol, 22, 31–36.
16. White NJ, Watt G, Bergqvist Y, Njelesani EK (1987). Parenteral chloroquine for treating falciparum malaria. J Infect Dis, 155, 192–201.
17. Tester-Dalderup CBM (1992). Antiprotozoal drugs: Antimalarial drugs. Meyler’s Side Effects of Drugs, 12th edn, pp 688–692.
18. Lange WR, Frankenfield DL, Moriarty-Sheehan M, Contoreggi CS, Frame JD et al. (1994). No evidence for chloroquine-associated retinopathy among missionaries on long-term malaria prophylaxis. Am J Trop Med Hyg, 51, 389–392.
19. Brokes DB (1966). Chloroquine psychosis. BMJ, I, 983.
20. Dornhorst AC, Robinson BF (1963). Chloroquine psychosis Lancet, i, 108.
21. Good MI, Shader RI (1977). Behavioral toxicity and equivocal suicide associated with chloroquine and its derivatives. Am J Psychiatry, 134, 798–801.
22. Fish DR, Espir MLE (1988). Convulsions associated with prophylactic antimalarial drugs: implications for people with epilepsy. BMJ, 297, 526–527.
23. Drysdale SF, Phillips-Howard PA, Behrens RH (1990). Proguanil, Chloroquine, and mouth ulcers. Lancet, 335, 164.
24. Ette EI, Brown-Awala EA, Essien EE (1987). Chloroquine elimination in humans: effect of lowdose cimetidine. J Clin Pharmacol, 27, 813–881.
Chloroquine is the most effective of the hundreds of 4-aminoquinolines synthesized and tested
during World War II as potential antimalarials. Structure–activity relationships demonstrated that
the chloro at the 8-position increased activity, whereas alkylation at C-3 and C-8 diminished
activity. The replacement of one of its N-ethyl groups with an hydroxyethyl produced hydroxychloroquine, a compound with reduced toxicity that is rarely used today except in cases of
rheumatoid arthritis.
Chloroquine used in the treatment of malaria and MDR-strains. It is a COVID19-related research product.
Chloroquine may be the drug of choice, but only in areas
where chloroquine-sensitive P. falciparum organisms are
present. Chloroquine prophylaxis is no longer effective
for travel to many regions. Daily atovaquone–proguanil
appears to be the first choice for chemoprophylaxis for
travel to areas of chloroquine resistance. Prophylactic
drugs, such as chloroquine or mefloquine, should be
started 2 to 4 weeks prior to travel and continued for
6 to 8 weeks after leaving the endemic areas. The
atovaquone–proguanil combination is the exception in
that it is started 1 to 2 days prior to departure and is continued 1 week after return.
Chloroquine (Aralen) is one of several 4-aminoquinoline
derivatives that display antimalarial activity.
Chloroquine is particularly effective against intraerythrocytic
forms because it is concentrated within the parasitized
erythrocyte. This preferential drug accumulation
appears to occur as a result of specific uptake
mechanisms in the parasite. Chloroquine appears to
work by intercalation with DNA, inhibition of heme
polymerase or by interaction with Ca++–calmodulinmediated
mechanisms. It also accumulates in the parasite’s
food vacuoles, where it inhibits peptide formation
and phospholipases, leading to parasite death.
ChEBI: An aminoquinoline that is quinoline which is substituted at position 4 by a [5-(diethylamino)pentan-2-yl]amino group at at position 7 by chlorine. It is used for the treatment of malaria, hepatic amoebiasis, lupus erythematosus, light-sensitive skin erupti
ns, and rheumatoid arthritis.
Aralen hcl;Aralin (diphosphate);Artrochin;Avloclor (diphosphate);Endamal;Erestol;Hiliopar;Instana;Lagaquin;Letaquine;Malaraquin;Malarex (diphosphate);Malariron (diphosphate);Malquin;Miniquine;Nivaquine b';Nivembin;Norolon;Pfizerquin;Resichin;Resochin (diphosphate);Rivoquin;Salestol;Scaniquine (diphosphate);Serviquin;Siragon.
Chloroquine, a 4-aminoquinoline derivative, was introduced in the
1940s for the treatment and prophylaxis of malaria. It was subsequently found to be
effective in higher and prolonged dosage in the treatment of lupus erythematosus,
rheumatoid arthritis and nephritis. In the early 1970s its use in these latter conditions was largely discontinued when it was found that prolonged daily
administration at high dosage was associated with cases of retinopathy resulting
from local deposition of the compound. Chloroquine however remains a valuable
drug. It can be used continuously at the dosages required for malaria prophylaxis
for as long as five years without risk of undue accumulation and it is included in
the WHO Model List of Essential Drugs for both its antimalarial and antiamoebic
activity.
(Reference: (WHTAC1) The Use of Essential Drugs, 2nd Report of the WHO Expert
Committee, 722, , 1985)
Chloroquine accumulates 300-fold in infected erythrocytes
and acts against the early erythrocytic stages of all four species
of Plasmodium that cause human malaria. It is also active
against the gametocytes of P. vivax, P. ovale and P. malariae,
but not against the hepatic stages or mature erythrocytic
schizonts
and merozoites.
Resistance of P. falciparum is widespread and has become a
major problem. The mechanism appears to be either decreased
uptake or increased efflux of the drug by the parasite,
or both.
Changes in genes encoding a P-glycoprotein homolog, Pfmdr1,
and another putative transporter, Pfcrt, are associated with resistance.
Reversal of resistance with, for example, verapamil or
probenecid has been demonstrated in experimental models, but
human trials have been disappointing. Chloroquine-resistant P.
vivax has been reported in South America and South East Asia.
A synthetic 4-aminoquinoline, formulated as the phosphate
or sulfate for oral administration and as the hydrochloride or
sulfate for parenteral use. The salts are soluble in water.
The absorption of chloroquine from the gastrointestinal
tract is rapid and complete. The drug is distributed
widely and is extensively bound to body tissues,
with the liver containing 500 times the blood concentration.
Such binding is reflected in a large volume of distribution
(Vd). Desethylchloroquine is the major
metabolite formed following hepatic metabolism, and
both the parent compound and its metabolites are
slowly eliminated by renal excretion.The half-life of the
drug is 6 to 7 days.
Oral absorption: 80–90%
Cmax 300 mg oral: 0.25 mg/L after 1–6 h
Plasma half-life: c. 9 days (mean)
Volume of distribution: 200 L/kg
Plasma protein binding: 50–70%
There is extensive tissue binding and a high affinity for melanin- containing tissues. Chloroquine is extensively metabolized to a biologically active monodesethyl derivative that forms about 20% of the plasma level of the drug. The mean elimination half-life results from an initial phase (3–6 days), a slow phase (12–14 days) and a terminal phase (40 days). Renal clearance is about 50% of the dose.
Cmax 300 mg oral: 0.25 mg/L after 1–6 h
Plasma half-life: c. 9 days (mean)
Volume of distribution: 200 L/kg
Plasma protein binding: 50–70%
There is extensive tissue binding and a high affinity for melanin- containing tissues. Chloroquine is extensively metabolized to a biologically active monodesethyl derivative that forms about 20% of the plasma level of the drug. The mean elimination half-life results from an initial phase (3–6 days), a slow phase (12–14 days) and a terminal phase (40 days). Renal clearance is about 50% of the dose.
Chloroquine is the drug of choice for preventing and treating acute forms of malaria
caused by P. vivax, P. malariae, P. ovale, as well as sensitive forms of P. falciparum. The
mechanism of its action is not completely clear, although there are several hypotheses
explaining its antimalarial activity. Chloroquine and its analogs inhibit synthesis of nucleic
acids of the parasite by affecting the matrix function of DNA. This happens by preliminary binding of the drug through hydrogen bonds with the purine fragments, and subsequent
introduction of the chloroquine molecule between the orderly arranged base pairs into the
spirals of the DNA of the parasite. Thus chloroquine prevents transcription and translation,
which significantly limits the synthesis of DNA and RNA in the parasite. The selective
toxicity of chloroquine in particular with respect to malarial plasmodia is also attributed to
the ability of the parasitized red blood cells to concentrate the drug in amounts approximately 25 times greater than in normal erythrocytes.
There is also a different hypothesis. Chloroquine has a high affinity for tissues of the parasite and is concentrated in its cytoplasm. As a weak base, it increases the pH of the intracellular lysosome and endosome. A more acidic medium in these organelles is needed for the parasite to affect mammalian cells. As a result, chloroquine inhibits growth and development of parasites.
Thus the main quality of chloroquine that exceeds all other antimalarial drug is its effect on erythrocytic schizonts (hematoschizotropic action). However, chloroquine also possesses amebicidal action. It has also been observed to have immunodepressive and antiarrhythmic properties.
It is used for all types of malaria, for chemotherapy, as well as for non-gastric amebiasis, and amebic abscesses of the liver. Synonyms of this drug are nivaquine, quingamine, delagil, resoquine, atroquine, and others.
There is also a different hypothesis. Chloroquine has a high affinity for tissues of the parasite and is concentrated in its cytoplasm. As a weak base, it increases the pH of the intracellular lysosome and endosome. A more acidic medium in these organelles is needed for the parasite to affect mammalian cells. As a result, chloroquine inhibits growth and development of parasites.
Thus the main quality of chloroquine that exceeds all other antimalarial drug is its effect on erythrocytic schizonts (hematoschizotropic action). However, chloroquine also possesses amebicidal action. It has also been observed to have immunodepressive and antiarrhythmic properties.
It is used for all types of malaria, for chemotherapy, as well as for non-gastric amebiasis, and amebic abscesses of the liver. Synonyms of this drug are nivaquine, quingamine, delagil, resoquine, atroquine, and others.
The drug is effective against all four types of malaria
with the exception of chloroquine-resistant P. falciparum.
Chloroquine destroys the blood stages of the infection
and therefore ameliorates the clinical symptoms
seen in P. malariae, P. vivax, P. ovale, and sensitive P. falciparum
forms of malaria. The disease will return in P.
vivax and P. ovale malaria, however, unless the liver
stages are sequentially treated with primaquine after
the administration of chloroquine. Chloroquine also can
be used prophylactically in areas where resistance does
not exist. In addition to its use as an antimalarial,
chloroquine has been used in the treatment of rheumatoid
arthritis and lupus erythematosus,
extraintestinal amebiasis, and photoallergic reactions.
Prophylaxis and treatment of all types of malaria
Hepatic amebiasis (in sequential combination with dehydroemetine)
A combination with azithromycin has been suggested for intermittent preventive treatment.
Hepatic amebiasis (in sequential combination with dehydroemetine)
A combination with azithromycin has been suggested for intermittent preventive treatment.
Dizziness, headache, itching (especially in darkskinned
people), skin rash, vomiting, and blurring of vision
may occur following low doses of chloroquine. In
higher dosages these symptoms are more common, and
exacerbation or unmasking of lupus erythematosus or
discoid lupus, as well as toxic effects in skin, blood, and
eyes can occur. Since the drug concentrates in melanincontaining
structures, prolonged administration of high
doses can lead to blindness. Chloroquine should not be
used in the presence of retinal or visual field changes.
Minor side effects such as dizziness, headache, rashes, nausea
and diarrhea are common. Pruritus occurs in up to 20%
of Africans taking chloroquine. Long-term treatment can
induce CNS effects and cumulative dosing over many years
may cause retinopathy. Rarely, photosensitization, tinnitus
and deafness have occurred.
Chloroquine, 7-chloro-4-(4-diethylamino-1-methylbutylamino)-quinoline (37.1.3), is made by reacting 4,7-dichloroquinoline (37.1.1.1) with 4-diethylamino- 1-methylbutylamine (37.1.1.2) at 180 °C.

In order to realize the synthesis, the necessary 4,7-dichloroquinoline (37.1.1.1) is prepared in several ways from 3-chloroaniline. One of these ways consists of reacting 3-chloroaniline with ethoxymethylenmalonic ester to make (3-choroanilino)-methylenemalonic ester (37.1.1.4), which then undergoes high-temperature heterocyclization to make the ethyl ester of 7-chloro-4-hydroxyquinolin-3-carboxylic acid (37.1.1.5). Hydrolyzing this with sodium hydroxide gives 7-chloro-4-hydroxyquinolin-3-decarboxylic acid (37.1.1.6), which when heated at 250–270 ° C is decarboxylated, forming 7-chloro-4-hydroxyquinoline (37.1.1.7). Treating this with phosphorus oxychloride gives one of the desired components for synthesis of chloroquine – 4,7-dichloroquinoline (37.1.1.1).
The second method of preparing of 4,7-dichloroquinoline (37.1.1.1) consists of reacting 3-chloroaniline with the diethyl ester of oxaloacetic acid in the presence of acetic acid to give the corresponding enamine (37.1.1.8), which when heated to 250 ° C undergoes heterocyclization to the ethyl ester of 7-chloro-4-hydrozyquinolin-2-carboxylic acid (37.1.1.9) accompanied with a small amount of 5-chloro-4-hydroxyquinolin-2-carboxylic acid (37.1.1.10), which is separated from the main product by crystallization from acetic acid. Alkaline hydrolysis of the ethyl ester of the 7-chloro-4-hydroxyquinolin-2-carboxylic acid (37.1.1.9) and subsequent high-temperature decarboxylation of the resulting acid (37.1.1.11) gives 7-chloro-4-hydroxyquinolin (37.1.1.7). Reacting this with phosphorus oxychloride using the scheme described above gives 4,7-dichloroquineoline (37.1.1.1).
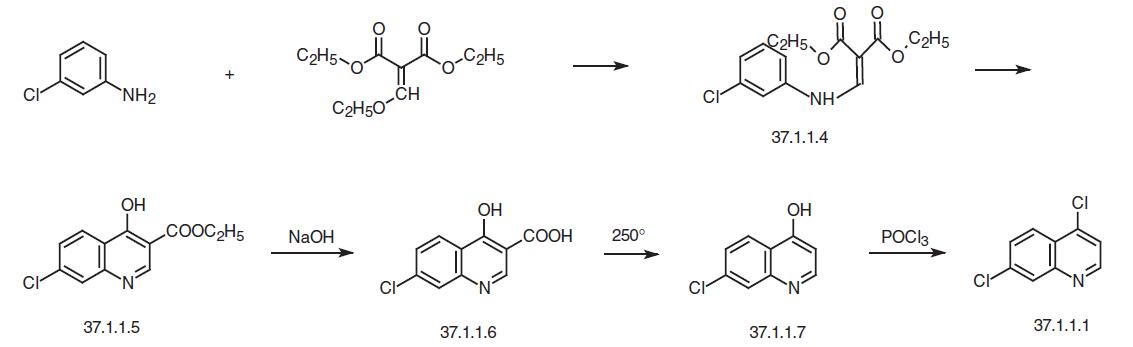
Finally, the third of the suggested variants for making 4,7-dichloroquinoline (37.1.1.1) consists of reacting 3-chloroaniline with the ethyl ester of formylacetic acid to make the enamine (37.1.1.12), which on heating directly cyclizes to 7-chloro-4-hydroxyquinoline (37.1.1.7). Reacting this with phophorus oxychloride according to the scheme already described gives 4,7-dichloroquinoline (37.1.1.1).
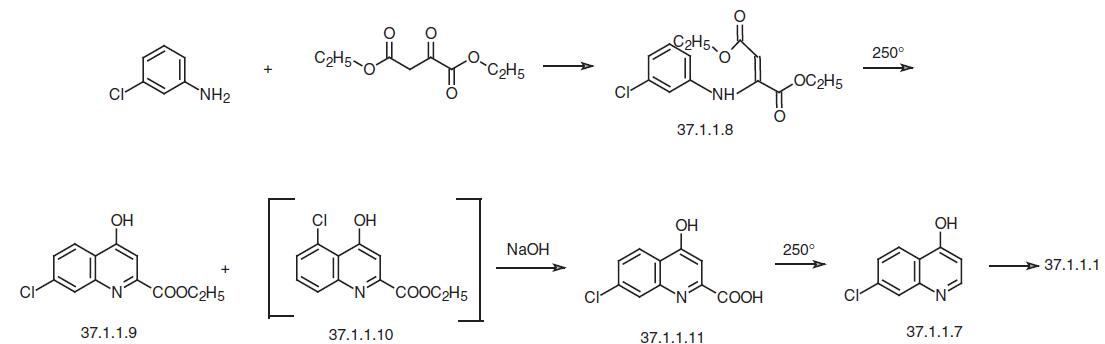
The second component necessary for synthesizing of the chloroquine is 4-diethylamino- 1-methylbutylamine (37.1.1.2), is also made in various ways. Alkylating acetoacetic ester with 2-diethylaminoethylchloride gives 2-diethylaminoethylacetoacetic acid ester (37.1.1.13), which upon acidic hydrolysis (using hydrochloric acid) and simultaneous decarboxylation makes 1-diethylamino-4-pentanone (37.1.1.14). Reductive amination of this compound with hydrogen and ammonia using Raney nickel as a catalyst gives 4-diethylamino-1-methylbutylamine (37.1.1.2).


In order to realize the synthesis, the necessary 4,7-dichloroquinoline (37.1.1.1) is prepared in several ways from 3-chloroaniline. One of these ways consists of reacting 3-chloroaniline with ethoxymethylenmalonic ester to make (3-choroanilino)-methylenemalonic ester (37.1.1.4), which then undergoes high-temperature heterocyclization to make the ethyl ester of 7-chloro-4-hydroxyquinolin-3-carboxylic acid (37.1.1.5). Hydrolyzing this with sodium hydroxide gives 7-chloro-4-hydroxyquinolin-3-decarboxylic acid (37.1.1.6), which when heated at 250–270 ° C is decarboxylated, forming 7-chloro-4-hydroxyquinoline (37.1.1.7). Treating this with phosphorus oxychloride gives one of the desired components for synthesis of chloroquine – 4,7-dichloroquinoline (37.1.1.1).
The second method of preparing of 4,7-dichloroquinoline (37.1.1.1) consists of reacting 3-chloroaniline with the diethyl ester of oxaloacetic acid in the presence of acetic acid to give the corresponding enamine (37.1.1.8), which when heated to 250 ° C undergoes heterocyclization to the ethyl ester of 7-chloro-4-hydrozyquinolin-2-carboxylic acid (37.1.1.9) accompanied with a small amount of 5-chloro-4-hydroxyquinolin-2-carboxylic acid (37.1.1.10), which is separated from the main product by crystallization from acetic acid. Alkaline hydrolysis of the ethyl ester of the 7-chloro-4-hydroxyquinolin-2-carboxylic acid (37.1.1.9) and subsequent high-temperature decarboxylation of the resulting acid (37.1.1.11) gives 7-chloro-4-hydroxyquinolin (37.1.1.7). Reacting this with phosphorus oxychloride using the scheme described above gives 4,7-dichloroquineoline (37.1.1.1).
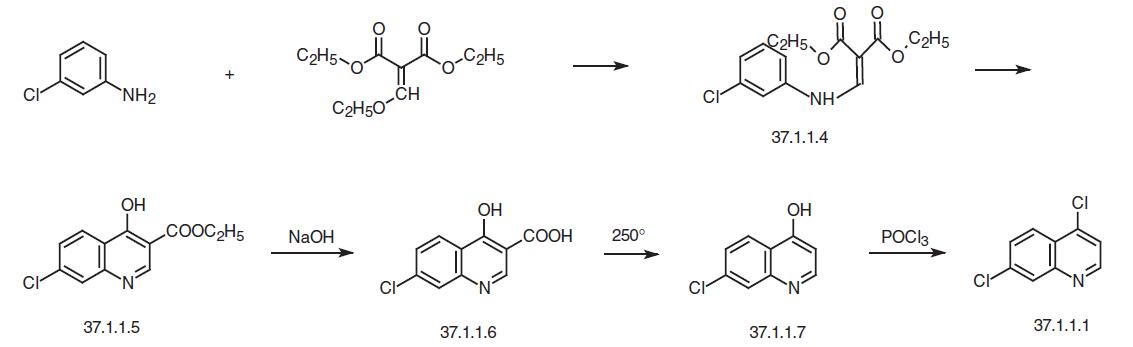
Finally, the third of the suggested variants for making 4,7-dichloroquinoline (37.1.1.1) consists of reacting 3-chloroaniline with the ethyl ester of formylacetic acid to make the enamine (37.1.1.12), which on heating directly cyclizes to 7-chloro-4-hydroxyquinoline (37.1.1.7). Reacting this with phophorus oxychloride according to the scheme already described gives 4,7-dichloroquinoline (37.1.1.1).
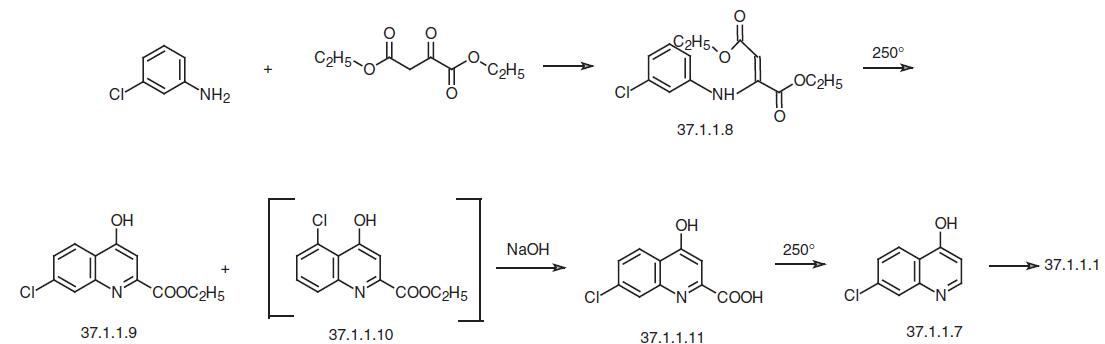
The second component necessary for synthesizing of the chloroquine is 4-diethylamino- 1-methylbutylamine (37.1.1.2), is also made in various ways. Alkylating acetoacetic ester with 2-diethylaminoethylchloride gives 2-diethylaminoethylacetoacetic acid ester (37.1.1.13), which upon acidic hydrolysis (using hydrochloric acid) and simultaneous decarboxylation makes 1-diethylamino-4-pentanone (37.1.1.14). Reductive amination of this compound with hydrogen and ammonia using Raney nickel as a catalyst gives 4-diethylamino-1-methylbutylamine (37.1.1.2).

Potentially hazardous interactions with other drugs
Anti-arrhythmics: increased risk of ventricular arrhythmias with amiodarone - avoid.
Antibacterials: increased risk of ventricular arrhythmias with moxifloxacin - avoid; concentration of praziquantel reduced - consider increasing praziquantel dose.
Anti-depressants: possible increased risk of ventricular arrhythmias with citalopram and escitalopram.
Antiepileptics: antagonism of anticonvulsant effect.
Antimalarials: increased risk of convulsions with mefloquine; avoid with artemether/lumefantrine.
Antipsychotics: increased risk of ventricular arrhythmias with droperidol - avoid.
Ciclosporin: increases ciclosporin concentration - increased risk of toxicity.
Cytotoxics: possible increased risk of ventricular arrhythmias with bosutinib, ceritinib and panobinostat.
Digoxin: possibly increased concentration of digoxin.
Lanthanum: absorption possibly reduced by lanthanum, give at least 2 hours apart.
Anti-arrhythmics: increased risk of ventricular arrhythmias with amiodarone - avoid.
Antibacterials: increased risk of ventricular arrhythmias with moxifloxacin - avoid; concentration of praziquantel reduced - consider increasing praziquantel dose.
Anti-depressants: possible increased risk of ventricular arrhythmias with citalopram and escitalopram.
Antiepileptics: antagonism of anticonvulsant effect.
Antimalarials: increased risk of convulsions with mefloquine; avoid with artemether/lumefantrine.
Antipsychotics: increased risk of ventricular arrhythmias with droperidol - avoid.
Ciclosporin: increases ciclosporin concentration - increased risk of toxicity.
Cytotoxics: possible increased risk of ventricular arrhythmias with bosutinib, ceritinib and panobinostat.
Digoxin: possibly increased concentration of digoxin.
Lanthanum: absorption possibly reduced by lanthanum, give at least 2 hours apart.
The exact mechanism of action of CQ and HCQ is not
completely understood but involves inhibition of DNA and
RNA polymerase. They are also direct myocardial depressants
that impair cardiac conduction through membrane stabilization.
It is unclear how they work in autoimmune diseases.
Chloroquine is extensively metabolised in the
liver, mainly to monodesethylchloroquine with
smaller amounts of bisdesethylchloroquine
(didesethylchloroquinine) and other metabolites being
formed. Monodesethylchloroquine has been reported to
have some activity against Plasmodium falciparum
Chloroquine and its metabolites are excreted in the urine, with about half of a dose appearing as unchanged drug and about 10% as the monodesethyl metabolite.
Chloroquine may be detected in urine for several months.
Chloroquine and its metabolites are excreted in the urine, with about half of a dose appearing as unchanged drug and about 10% as the monodesethyl metabolite.
Chloroquine may be detected in urine for several months.
Preparation Products And Raw materials
Raw materials
Preparation Products
Related articles
Related Product Information
- Hydroxychloroquine sulfate
- Mepacrine hydrochloride
- Aminoquinol
- QUINACRINE MUSTARD DIHYDROCHLORIDE
- Chloroquine diphosphate
- Hydroxychloroquine
- Chloroquine sulphate BP2000,chloroquine sulfate
- CHLOROQUINE
- 4,7-DICHLOROQUINOLINE CHLOROQUINE PHOSPHATE
- Didesethyl Chloroquine,Didesethyl Chloroquine Hydrochloride,BISDESETHYL CHLOROQUINE
1of4
PROMPT×
PROMPT
The What'sApp is temporarily not supported in mainland China
The What'sApp is temporarily not supported in mainland China
Cancel
Determine