Methyl oleate: synthesis and its application research
Introduction
Methyl oleate is a fatty acid methyl ester with a cis monounsaturated structure, of which Chemical name is 9-octadecenoic acid methyl ester. Methyl oleate appears as a clear and transparent oily liquid at room temperature (Figure 1). Its physical parameters include: a density of 0.8739 g/cm³(20℃), a melting point of -19.9℃, a boiling point at atmospheric pressure of 351.4℃ (accompanied by thermal decomposition), a vacuum distillation range of 168-218.5℃/10mmHg, and a refractive index of 1.452-1.454. Methyl oleate exhibits typical amphiphilic molecular behavior, which is immiscible with aqueous systems (logP≈7.3), but can be miscible with alcohols, ethers, and hydrocarbon solvents in any proportion. It is relatively stable thermodynamically, but is prone to oxidative degradation during long-term storage (the peroxide value increases linearly with time), especially under light or metal ion catalysis conditions, where the double bond region is prone to free radical chain reactions to produce hydroperoxide derivatives.
Optimization of methyl oleate synthesis
Enzymatic esterification
Esterification reactions were performed under solvent-free conditions on a 50 mL scale in baffled Erlenmeyer flasks. The molar ratio of methanol to fatty acid was 1:1, 1.5:1 or 2:1 (depending on specific reaction) and the lipase dose was 0.25, 0.50 or 0.75 wt% (depending on specific reaction). Reactions were incubated in a shaking incubator at 55◦C, 170 rpm, and samples were taken regularly over a period of 48 h. Reaction progress was monitored by titration of the residual free fatty acid. When the conversion took place in absence of water (to calculate the kinetic constants of the forward reaction (esterification) in absence of the backward reaction), the reaction was performed in the presence of molecular sieves 3 Å, previously activated by drying at 250◦C for 16 h.
Two different ratios of methanol/oleic acid (1:1 and 1.5:1) and three enzyme loadings (0.25, 0.50 and 0.75 wt%) were tested to optimize the synthesis of methyl oleate.
The results show that a maximum oleic acid conversion of 74% could be obtained with an equimolar mixture of both substrates, while the addition of methanol in 50% molar excess was necessary to increase the conversion to 99%. As expected, the higher the enzyme loading, the higher the initial reaction rate, but maximum equilibrium conversions were already reached after 24 h for all three lipase doses. The conversion value obtained in the present test is in line with those reported in literature for direct esterification of oleic acid and methanol under solvent-free conditions. Nguyen and co-workers optimized the solvent-free reaction catalyzed by the liquid lipase Eversa Transform by using response surface methodology. They obtained a conversion of 96.7% after 2.5 h, using the optimized conditions determined by the model: methanol to oleic acid molar ratio 3.33:1, lipase loading of 12%, temperature 35.3℃ and water removal via addition of a super adsorbent polymer. Pang and co-workers used wrinkled silica nanoparticles as a carrier to immobilize Candida rugosa lipase and use it as catalyst for the reaction, obtaining only 84% conversion. The large excess of methanol to oleic acid (20:1 molar ratio) used in the reaction could have been the cause for lower conversion values, since high amounts of methanol are reported to decrease reaction rates and lower product yields in lipase-catalyzed (trans) esterification. Since esterification is an equilibrium reaction, removal of the water formed as by-product can help in shifting that equilibrium towards the product side. Various technologies for water removal are available, such as addition of molecular sieves, bubbling inert gas (e.g. N2), performing the reaction under vacuum to evaporate water at reaction temperature (< 100℃) or pervaporation. Choosing one or the other depends on the properties of reaction components. In addition, it is essential to know the reaction composition at equilibrium when by-product water is still present in the system. To this end, the reaction between oleic acid and methanol at a molar ratio of alcohol/acid of 1.5 was also performed without addition of molecular sieves. The conversion curve in the reaction without molecular sieves matches the reaction with molecular sieves well, showing an almost negligible, but small difference. This indicates that water removal was able to affect the equilibrium only slightly.[1]
Application research
1.Effects of methyl oleate and microparticle enhanced cultivation on echinocandin B fermentation titer
Echinocandin B (ECB) is a key precursor of antifungal agent Anidulafungin, which has demonstrated clinical efficacy in patients with invasive candidiasis. In this study, the effects of microparticle-enhanced cultivation and methyl oleate on echinocandin B fermentation titer were investigated. The methyl oleate was the best carbon source; it could not only make the cells maintainin the optimal mycelial morphology, but also accumulatemore important precursor amino acid L-threonine. Besides,it was concluded that mycelium pellet was preferred for ECB production, and the addition of talcum powder could further improve the interior structure of the pellets, and the highest ECB titer could reach 3148±100 mg/L. The results showed that the titer was significantly influenced by the morphological type of mycelium, and mycelium pellet was beneficial to improve the titer of this secondary metabolism. First, different carbon sources were chosen for the fermentation, and methyl oleate achieved the highest echinocand in B titer of 2133 ± 50 mg/L, which was two times higher than that of the mannitol. The study further investigated the metabolic process of the fermentation, and the results showed that L-threonine concentration inside the cell could reach 275 mg/L at 168 h with methyl oleate, about 2.5 times higher than that of the mannitol. Therefore, L-threonine may be a key precursor of echinocandin B. In the end, a new method of adding microparticles for improving the mycelial morphology was used, and the addition of talcum powder (20 g/L, diameter of 45 µm) could make the maximum titer of echinocandin B reach 3148 ± 100 mg/L.[2]
2. Diffusion of methyl oleate in hierarchical micro-/ mesoporous TS-1-based catalysts probed by PFG NMR spectroscopy
Pulsed field gradient (PFG) NMR is successfully applied to trace the diffusion of methyl oleate (MO) inside the mesopores of hierarchically structured titanium silicalite-1 (TS-1)-based catalysts. Introduction of mesoporosity by post-synthetic treatment of initially microporous TS-1 provides additional active surface to improve catalytic activity in the epoxidation of methyl oleate. The present study provides experimental evidence of the accessibility of mesopores for methyl oleate resulting from alkaline treatment of TS-1. The self-diffusion coefficients of methyl oleate inside the pores of hierarchically structured TS-1 catalysts are up to two orders of magnitude lower compared to the values in the bulk liquid phase. Additionally, the methodological capability of PFG NMR for measuring self-diffusion coefficients of long-chain hydrocarbons (up to C19) confined to narrow mesopores of catalytically active is demonstrated for the first time.
Finally, the presented diffusion data demonstrate the feasibility of application of the PFG NMR technique for the direct determination of diffusion coefficients of high molecular weight long-chain hydrocarbons and oxygen-functionalized derivatives, such as methyl oleate, in catalysts with hierarchically structured pore systems.[3]
3. Methyl oleate modulates LIP2 expression in the lipolytic yeast Yarrowia lipolytica
Methyl oleate was used as a primary carbon source and as an alternative inducer for the production of an extracellular lipase, Lip2, in Y. lipolytica strain LgX64.81 grown in a 20-1 bioreactor. The lipase-encoding gene, LIP2, was investigated during culture on methyl oleate using a pLIP2–LacZ reporter fusion and researchers provide evidence for the involvement of methyl oleate in its regulation.
In conclusion, the lipase activity in the culture supernatant obtained in these conditions was equal to 1300 U ml )1 which is in accordance with the yield obtained previously on olive oil media. In addition,Patrick and his coworkers demonstrate in this paper that methyl oleate influenced LIP2 induction. The maximal induction was obtained in the presence of low methyl oleate concentration.[4]
References
[1] Martinez-Garcia M, Van Hecke W, Peeters H, et al. Methyl oleate for plant protection products formulations: Enzymatic synthesis, reaction kinetics and application testing. J Biotechnol. 2024;379:78-86. doi:10.1016/j.jbiotec.2023.12.004
[2] Niu K, Wu XP, Hu XL, et al. Effects of methyl oleate and microparticle-enhanced cultivation on echinocandin B fermentation titer. Bioprocess Biosyst Eng. 2020;43(11):2009-2015. doi:10.1007/s00449-020-02389-3
[3] Dvoyashkin M, Wilde N, Haase J, Gläser R. Diffusion of methyl oleate in hierarchical micro-/mesoporous TS-1-based catalysts probed by PFG NMR spectroscopy [published correction appears in RSC Adv. 2018 Nov 30;8(70):40060. doi: 10.1039/c8ra90098a.]. RSC Adv. 2018;8(68):38941-38944. Published 2018 Nov 20. doi:10.1039/c8ra07434h
[4] Fickers P, Destain J, Thonart P. Methyl oleate modulates LIP2 expression in the lipolytic yeast Yarrowia lipolytica. Biotechnol Lett. 2005;27(22):1751-1754. doi:10.1007/s10529-005-3547-2
You may like
Lastest Price from Methyl Oleate manufacturers
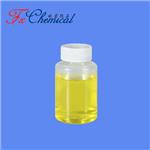
US $0.00/KG2025-04-21
- CAS:
- 112-62-9
- Min. Order:
- 25KG
- Purity:
- 98%min
- Supply Ability:
- 30tons/month
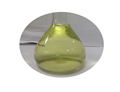
US $10.00/kg2025-04-21
- CAS:
- 112-62-9
- Min. Order:
- 1kg
- Purity:
- 99.5%
- Supply Ability:
- 100 TON