Dicyclohexylcarbodiimide: A Systematic Review of its Applications in Organic Chemistry
General Description
Dicyclohexylcarbodiimide is a powerful dehydrating agent commonly used for the preparation of amides, esters, and anhydrides; used with DMSO for the mild oxidation of alcohols to ketones; used in the dehydrative conversion of primary amides to nitriles, 𝛽-hydroxy ketones to 𝛼,𝛽-unsaturated ketones, and can effect the stereochemical inversion of secondary alcohols. Dicyclohexylcarbodiimide is an acute skin irritant in susceptible individuals. Because of its low melting point, it is conveniently handled as a liquid by gentle warming of the reagent container. It should be handled with gloves in a fume hood, and stored under anhydrous conditions.
Figure 1. Properties of Dicyclohexylcarbodiimide
Applications in Organic Reactions
Amide Formation
Figure 2. Amide Formation
Since the initial reports, dicyclohexylcarbodiimide has become the most common reagent in peptide synthesis and in other amide bond forming reactions of primary and secondary amines with carboxylic acids. The mechanism is considered to be well understood.
Typically, dicyclohexylcarbodiimide (1.1 equiv) is added to a concentrated solution (0.1–1.0 M) of the carboxylic acid (1.0 equiv), amine (1.0 equiv), and catalyst (when used) in methylene chloride or acetonitrile at 0 ◦C. The hydrated dicyclohexylcarbodiimide adduct, dicyclohexylurea (DCU), quickly precipitates and the reaction is generally complete within 1 h at rt. The solvents THF and DMF can be used, but are reported to reduce reaction rates and encourage the formation of the N-acylurea side product, as well as increasing racemization in chiral carboxylic acids. If the amine is initially present as the salt (i.e. amine hydrochloride), it may be neutralized by adding 1 equiv of Diisopropylethylamine prior to adding dicyclohexylcarbodiimide; however, the addition of tertiary amines (particularly Triethylamine) can facilitate N-acylurea formation and racemization. Racemization occurs via the formation of an oxazalone intermediate. The addition of coupling agents (acylation catalysts) such as 1-Hydroxybenzotriazole (HOBt), 1-hydroxy-7-azabenzotriazole (HOAt), N-Hydroxysuccinimide (HOSu), and 3-hydroxy-3,4-dihydro-1,2,3-benzotriazin-4-one can generally ameliorate both problems. These additives are required for the coupling of sterically hindered components, or when the amine is weakly nucleophilic.1
Another potential problem with dicyclohexylcarbodiimide is that at the completion of the reaction some DCU remains in solution with the product, necessitating additional purification. Watersoluble carbodiimide derivatives such as 1-Cyclohexyl-3-(2-morpholinoethyl)carbodiimide Metho-p-toluenesulfonate and 1-Ethyl-3-(3′-dimethylaminopropyl)carbodiimide Hydrochloride (EDCI) obviate this problem, as they are removed by a simple extraction. Many newer coupling agents have been developed for peptide synthesis and other acylation reactions. These include Benzotriazol-1-yloxytris(dimethylamino)phosphonium Hexafluorophosphate (BOP), O-Benzotriazol-1-yl-N,N,N′,N′-tetramethyluronium Hexafluorophosphate (HBTU), Bis(2-oxo-3-oxazolidinyl)phosphinic Chloride (BOP-Cl), and (1H-1,2,3-benzotriazol-1-yloxy)tris(pyrrolidino)phosphonium hexafluorophosphate (PyBOP). In addition to linear and polymeric amides, lactams of various ring sizes have been synthesized using these methods.2
Ester and Thioester Formation
These reactions occur through the same O-acylurea or anhydride active intermediate as in the amide coupling reactions, and the discussion of associated problems applies here as well. In general, alkyl and (particularly)aryl thiols can be efficiently coupled to carboxylic acids using dicyclohexylcarbodiimide. Reactions of primary and secondary alcohols proceed reliably, but require the presence of an acylation catalyst. This is usually 4-Dimethylaminopyridine (DMAP), but others have been used including 4-pyrrolidinopyridine and pyridine (solvent) with catalytic p-Toluenesulfonic Acid. The acylation of more hindered alcohols often results in reduced yields; however, even t-butanol can be acylated, providing a useful route to t-butyl esters. Various other carbodiimide derivatives have also been used in the preparation of esters. As with amides, which are not limited to intermolecular reactions, a wide variety of lactones can also be synthesized.3
Anhydride Formation
Figure 3. Anhydride Formation
Among other anhydride-forming reagents, including Acetic Anhydride, Trifluoroacetic Anhydride, and Phosphorus(V) Oxide, dicyclohexylcarbodiimide is one of the simplest, mildest, and most effective reagents for the preparation of symmetrical anhydrides, including formic anhydride, which is useful in the preparation of formamides. Anhydride formation is associated with each of the reaction types involving carboxylic acids. The anhydride is often the reactive species, to the extent that it competes with the O-acylurea or covalent catalyst adduct.4
Moffatt Oxidation
Figure 4. Moffatt Oxidation
The oxidation of primary and secondary alcohols to aldehydes and ketones, respectively, can be carried out by reaction with DMSO activated by dicyclohexylcarbodiimide. In comparison to the many metal-mediated oxidative reactions, the Moffatt oxidation is carried out under very mild conditions, and has found widespread use for reactions in the presence of sensitive functional groups. In addition, over-oxidation of aldehydes to form carboxylic acids is not observed. Under typical reaction conditions, a solution of the alcohol (1 equiv), dicyclohexylcarbodiimide (3 equiv), and a proton source (pyridinium trifluoroacetate) (0.5 equiv) is stirred in DMSO or DMSO/benzene overnight at rt. After quenching the reaction with aqueous acetic acid and removing DCU by filtration, the product can be isolated by extraction. In modifications of this reaction, dicyclohexylcarbodiimide can be replaced by other DMSO activating agents including acetic anhydride, trifluoroacetic anhydride, and oxalyl chloride.5
Guanidine Formation
Figure 5. Guanidine Formation
Because of the electrophilicity of carbodiimide, the nucleophilic addition of amines can lead the dicyclohexylcarbodiimide to the formation of guanidines. Using 𝛼-amino amides, which are derived from L-pipecolic acid, L-proline, or L-ramipril, chiral guanidines can be synthesized. The lithiated amino amide was formed in the presence of n-BuLi, followed by the addition of dicyclohexylcarbodiimide to furnish the guanidine. The X-ray crystallographic analysis indicated that the guanidine formed two H-bonds, one intermolecularly and one intramolecularly. In addition, the C2-symmetric chiral bisguanidines could be obtained with enantiopure or achiral linkers.6
The dicyclohexylcarbodiimide-derived chiral guanidine serves as an efficient organocatalyst, promoting the Michael addition of 𝛽-ketoesters to nitroolefins with excellent enantioselectivities and distereoselectivities. The guanidine or guanidium organocatalysts were found to affect a series of asymmetric transformations, including the inverse-electron-demand hetero-Diels–Alder reaction of chalcones with azlactones, the direct vinylogous Michael reaction of 𝛼,𝛽-unsaturated 𝛾-butyrolactam with alkylidene malonate, intramolecular aza-Michael reaction, Mannich-type reaction, and kinetic resolution reactions.7
Achiral guanidines can be synthesized through the addition of amines to carbodiimides. Yb(OTf)3 was found to efficiently catalyze the addition of primary aromatic amines and secondary cyclic amines to carbodiimides under solvent-free conditions. Using only 0.5 mol % catalyst loading, the reaction could be completed within 1 h at room temperature with primary aromatic amine substrate, affording the N,N′,N′-trisubstituted guanidine in excellent yield. Alternatively, the reaction between dicyclohexylcarbodiimide and 1,2-diarylhydrazine also provided a guanidine. In this reaction, guanidine and azo compounds were obtained in the presence of LiCH2TMS as a catalyst. Cleavage of the N–N bond in 1,2-diarylhydrazine was attributed to an intramolecular proton shift.8
Heterocyclization Reaction
dicyclohexylcarbodiimide has frequently been used both as a reagent and as a reactant in the synthesis of heterocycles. For example, dicyclohexylcarbodiimide-mediated cyclodesulfurative annulation reactions have been used to synthesize guanosinetype nucleotide analogs.
Figure 6. Heterocyclization Reactions
Preparation of Dicyclohexylcarbodiimide
Figure 6. Preparation of Dicyclohexylcarbodiimide
The practical and sophisticated method of the chemical synthesis process of dicyclohexylcarbodiimide had the thiocarbamide method in the prior art, urea method photoreactive gas method.The thiocarbamide method has two route of synthesis: first method is that Weith in 1873 at first proposes, and remains preparation dicyclohexylcarbodiimide and other carbodiimide one of method preferably at present, and yield can reach 86%. But the compound of mercury uses contaminate environment, and therefore only the appropriate experimental chamber prepares on a small quantity. Second method is to use the hypochlorite oxidation depriving hydrogen sulphide instead, and raw material is easy to get, and production cost is low, and yield can reach 90%, is the method for China industrial production dicyclohexylcarbodiimide.
References
1. Bodanszky, M. Peptide Chemistry: A Practical Textbook; Springer: New York, 1988.
2. Tanner, D.; Somfai, P., Tetrahedron 1988, 44, 613.
3. Grunwell, J. R.; Foerst, D. L., Synth. Commun. 1976, 6, 453.
4. Rammler, D. H.; Khorana, H. G., J. Am. Chem. Soc. 1963, 85, 1997.
5. Albright, J. D.; Goldman, L., J. Org. Chem. 1965, 30, 1107.
6. Yu, Z. P.; Liu, X. H.; Zhou, L.; Lin, L. L.; Feng, X. M., Angew. Chem. Int. Ed. 2009, 48, 5195.
7. Dong, S. X.; Liu, X. H.; Chen, X. H.; Mei, F.; Zhang, Y. L.; Gao, B.; Lin, L. L.; Feng, X. M., J. Am. Chem. Soc. 2010, 132, 10650.
8. Yang, Y.; Dong, S. X.; Liu, X. H.; Lin, L. L.; Feng, X. M., Chem. Commun. 2012, 48, 5040.
You may like
Related articles And Qustion
See also
Lastest Price from Dicyclohexylcarbodiimide manufacturers
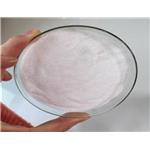
US $1.00/g2025-04-19
- CAS:
- 538-75-0
- Min. Order:
- 1g
- Purity:
- 99%
- Supply Ability:
- 100kg
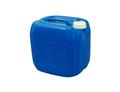
US $10.00/kg2025-04-19
- CAS:
- 538-75-0
- Min. Order:
- 1kg
- Purity:
- 99%
- Supply Ability:
- 200tons