Uses
neurotransmitter (ester of choline and acetic acid)
Description
Acetylcholine is a choline molecule that has been acetylated at the oxygen atom. Because
of the presence of a highly polar, charged ammonium group, acetylcholine does not penetrate lipid membranes. Because of this, when the drug is introduced externally, it remains
in the extracellular space and does not pass through the blood–brain barrier.
Description
Acetylcholine
is stored in vesicles in the presynaptic neuron. These
fuse with presynaptic membrane upon stimulation by a
nerve signal, thus, generating a pulse of neurotransmitter,
which diffuses across the membrane. Acetylcholine may
either bind reversibly to one of two different types of
acetylcholine receptors on the postsynaptic membrane
or be destroyed by the acetylcholine-hydrolyzing enzyme,
acetylcholinesterase.
Definition
ChEBI: Acetylcholine is actylcholine is an ester of acetic acid and choline, which acts as a neurotransmitter. It has a role as a vasodilator agent, a muscarinic agonist, a hormone, a human metabolite, a mouse metabolite and a neurotransmitter. It is an acetate ester and an acylcholine.
Brand name
Miochol (Novartis).
Biological Functions
The discovery that ACh was a transmitter in the peripheral
nervous system formed the basis for the theory of
neurotransmission. ACh is also a neurotransmitter in
the mammalian brain; however, only a few cholinergic
tracts have been clearly delineated.ACh is an excitatory
neurotransmitter in the mammalian CNS.There is good
evidence that ACh (among other neurotransmitters) is
decreased in certain cognitive disorders, such as
Alzheimer’s disease.
Biological Activity
Acetylcholine is a neurotransmitter found in the nervous systems of all animals. It is involved in the control of functions as diverse as locomotion, digestion, cardiac rate, “fight and flight” responses, secretion, learning and memory. Cholinergic dysfunction is associated with neuromuscular diseases such as myasthenia gravis and neurodegenerative disorders such as Alzheimer disease.
Studies of acetylcholine and cholinergic neurotransmission have played a key role in the development of nearly all aspects of our current understanding of chemical synaptic transmission. In the early part of the twentieth century, pioneering physiological and neurochemical experiments resulted in establishing the principle that release of neuroactive compounds, such as acetylcholine, on to effector cells or other neurons forms the basis of most types of intercellular communication. In these early studies, application of acetylcholine could mimic the effects of nerve stimulation on muscle contraction, the rate of heart beating, etc., and the compound was thus identified as the first neurotransmitter substance. It was also noted that not all nerves released acetylcholine when stimulated, thus indicating specificity for the type of neurotransmitter substances present in particular neurons. Pharmacological work identified compounds, extracted primarily from plants, which differentially blocked the action of acetylcholine on particular types of effector cells, leading to the concept of receptor specificity. The quantal nature of neurotransmitter release was also first appreciated at cholinergic neuromuscular junctions. Finally, the nicotinic acetylcholine receptor was the first ligand-gated ion channel to have its amino acid sequence established.
Acetylcholine is a simple ester of the quaternary amino alcohol choline and acetic acid. Acetylcholine is positively charged at physiological pH, is freely soluble in water (usually supplied as a bromide or chloride salt) and is subject to rapid hydrolysis in solution by heat or alkali. Nuclear magnetic resonance studies indicate considerable flexibility of the molecule in solution, and different conformations are thought to bind to different types of acetylcholine receptor.
Mechanism of action
Acetylcholine functions primarily as a chemical neurotransmitter in the nervous systems of all animals. When a cholinergic neuron is excited, it releases transmitter into the synaptic cleft where it can bind to a number of different receptor proteins. The receptors for acetylcholine can be classified into two general categories based primarily on the actions of different plant alkaloids that affect their function: nicotinic (nicotine binding) or muscarinic (muscarine binding). Several different subtypes for each of these general receptor classes have been characterized. The receptor binding event can be transduced into opening of cationic or anionic ion channels or coupled to some other metabolic signal such as phospholipid turnover rates or activation of second-messenger systems. Both inhibitory or, more commonly, excitatory responses are induced in the neurons or effector cells which receive the neurotransmitter signal, making acetylcholine-mediated neurotransmission particularly versatile.
In addition to the ubiquitous presence of acetylcholine in the nervous systems of all animals, it is also found in a limited number of plants, bacteria, fungi and protozoa. This widespread distribution in a variety of species most likely indicates the appearance of acetylcholine-metabolizing proteins fairly early in evolutionary history. In vertebrates, acetylcholine is also found in non-neuronal tissues such as primate placenta and sperm where its functional role, if any, remains unknown.
Clinical Use
The cholinergic system was the first neurotransmitter system shown to have a role in wakefulness and initiation
of REM sleep. Because of the poor penetration of the cholinergic drugs into the CNS, the role of this
system in sleep has relied on animal studies using microinjection into the brain, primarily in the area of the
dorsal pontine tegmentum. Acetylcholine, cholinergic agonists (e.g., arecoline or bethanechol), and
cholinesterase inhibitors are effective in the initiation of REM sleep from NREM sleep after microinjection.
Conversely, administration of anticholinergic drugs (e.g., atropine or scopolamine) hinders the transition to
REM sleep. Increase in the rate of discharge of these cholinergic cells (that activate the thalamus, cerebral
cortex, and hippocampus) during REM sleep parallel the same pattern seen with arousal and alertness.
Synthesis
Acetylcholine, 2-acetoxy-N,N,N-trimethylethyl ammonium chloride
(13.1.2), is easily synthesized in a number of different ways. For example, 2-chloroethanol
is reacted with trimethylamine, and the resulting N,N,N-trimethylethyl-2-ethanolamine
hydrochloride (13.1.1), also called choline, is acetylated by acetic acid anhydride or
acetylchloride, giving acetylcholine (13.1.2). A second method consists of reacting
trimethylamine with ethylene oxide, giving N,N,N-trimethylethyl-2-ethanolamine hydroxide (13.1.3), which upon reaction with hydrogen chloride changes into the hydrochloride
(13.1.1), which is further acetylated in the manner described above. Finally, acetylcholine
is also formed by reacting 2-chloroethanol acetate with trimethylamine [1¨C7].
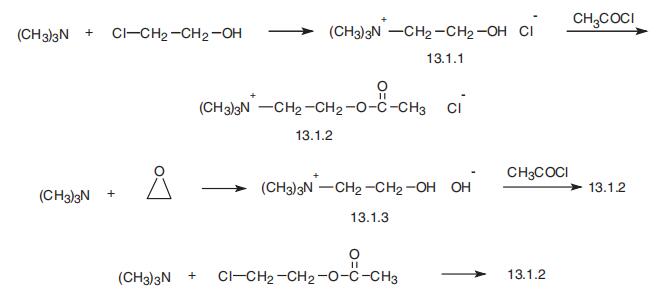
Environmental Fate
Cholinergic agents can increase the acetylcholine level at the
synaptic junction and cause rapid firing of the postsynaptic
membrane. Antiacetylcholinesterase agents block the acetylcholinesterase
enzyme and thus increase the acetylcholine level
in the synapse causing rapid firing of the postsynaptic
membrane.
Metabolism
Acetylcholine in the synapse can bind with cholinergic receptors on the postsynaptic or presynaptic membranes to produce a response. Free
acetylcholine that is not bound to a receptor is hydrolyzed by AChE. This hydrolysis is the physiologic mechanism for terminating the action of
acetylcholine. Enough AChE is present in the synapse to hydrolyze approximately 3 × 108
molecules of acetylcholine in 1 millisecond; thus,
adequate enzyme activity exists to hydrolyze all the acetylcholine (~3 × 106
molecules) released by one action potential. A number of useful
therapeutic cholinomimetic agents have been developed based on the ability of the compounds to inhibit AChE; these agents are addressed later
in this chapter.