Somatostatin: Insights into Hormone Function, Receptor Architecture, and Clinical Utility
Somatostatin is a hormone that regulates a variety of bodily functions by hindering the release of other hormones, the activity of your gastrointestinal tract and the rapid reproduction of cells. It is widely distributed throughout the human central nervous system (CNS) and peripheral tissues.
Somatostatin, a Presynaptic Modulator
The cyclic tetradecapeptide somatostatin was initially identified as a hypothalamic peptide that regulates growth hormone secretion from cells of the anterior pituitary gland. Soon after, data were provided showing that somatostatin is widely expressed in the central nervous system (CNS), where it plays a main role as regulator of neuronal functions. Here, the peptide exists in two main biologically active forms, the somatostatin-14 (SRIF-14), which is the peptide firstly discovered and the amino-terminal extended form, we refer to as the somatostatin-28 (SRIF-28). In mammals, somatostatin originates from a single gene that encodes for preprosomatostatin (116 amino acids) which is cleaved into prosomatostatin (96 amino acids) from which SRIF-14 and SRIF-28 originate. Somatostatin mediates its actions through a family of G protein-coupled receptors (GPCRs) having a structural distribution in cell membranes. These receptors are typified by seven transmembrane domains that couple either to inhibitory or facilitatory G proteins. Five genes encoding the distinct somatostatin receptor subtypes, we refer to as sst1-sst5 receptors, have been decoded in humans and other species. Among these structures, the carboxy-terminal tail of the sst2 receptor undergoes alternative splicing further yielding to other two isoforms, namely the sst2A and sst2B receptors. The two natural active forms of somatostatin (namely SRIF-14 and SRIF-28) have comparable affinities towards the different receptor subtypes and cannot be used to pharmacologically discriminate them.[1]
The study of the role of the Na+ ions in controlling the release of somatostatin, however, unveiled a more complicated scenario than expected. Although the secretion of somatostatin from cortical nerve endings in both resting and depolarized conditions was strictly dependent on the membrane Na+ gradient, the correlation linking the somatostatin release and the [Na+]out shifts from positive to negative depending on the nature of the stimulus applied. The [Na+]out was found to be positively associated with somatostatin exocytosis elicited by a mild depolarizing stimulus, but the correlation became negative when analysing the release of the peptide in resting conditions. In the latter case, lowering the extracellular concentration of Na+ (but not increasing it) unveiled a Ca2+-insensitive, non-exocytotic release of somatostatin. To the best of our knowledge, the mechanism(s) accounting for the low Na+-induced releasing activity remains so far unknown, also because the involvement of an uptake carrier working in the reverse mode (as proposed for classic neurotransmitters) seems unlike, mainly because of the lack of reuptake system(s) specific for somatostatin in nerve terminals. Interestingly, beside Na+ ions, also protons largely influence the efficiency of peptide outflow. Alkalinization (but not acidification) of the external milieu was shown to increase somatostatin release through a cascade of events leading to a conventional exocytosis but also to a concomitant outflow that is independent from external Ca2+ ions.
As far as somatostatin is concerned, the data in the literature unveiled a more complex scenario. In 1998, Dournaud and colleagues investigated the distribution of the sst2 receptors in selected regions of the CNS and correlated this parameter to the distribution of the somatostatin-containing terminals. The study was limited to the sst2 receptors, which account for a relevant percentage of the somatostatin receptors in the CNS. It focused on the relative distribution of the somatostatin-containing axons and of the sst2 receptors expressing neurons, providing an important picture of the central somatostatinergic innervation. The authors described two main patterns of immunostaining, the first showing a clear somato-dendritic distribution, the second typified by a diffuse immunopositivity within the tissue. Interestingly, the sst2 receptor labelling in the somato-dendritic regions was differently scattered in the neuronal compartment depending on the relative distribution of somatostatin in axon terminals. In those regions where the local somatostatin immunoreactivity was high, the sst2 receptors were preferentially intracellular and only a small portion of them was associated with plasma membranes.
Somatostatin receptors: From signaling to clinical practice
Somatostatin is a naturally occurring inhibitory polypeptide hormone first isolated from the sheep hypothalamus (Brazeau et al., 1973). Somatostatin has a broad range of biological actions that include the regulation of neurotransmission and secretion and the inhibition of the release of growth hormone (GH), thyroid-stimulating hormone (TSH), gastrointestinal (GI) hormones, pancreatic enzymes and neuropeptides. Increased understanding of SSTR signaling and function has highlighted the antiproliferative role of somatostatin and provided the rationale for the design of novel somatostatin analogs and therapeutics. This review addresses the following points: (i) antisecretory action of somatostatin and the antiproliferative signaling cascades of each SSTR; (ii) somatostatin analogs and their clinical use; (iii) resistance to somatostatin analogs; and (iv) side effects associated with somatostatin analogs.[2]
The effect of somatostatin on hormone transcription is less clear. Initial studies did not find changes in GH mRNA levels after somatostatin administration, supporting the hypothesis that somatostatin suppresses GH secretion by blocking exocytosis rather than transcription. However, studies performed in vitro and in tumors from patients with acromegaly who were preoperatively treated with somatostatin analogs revealed reduced GH transcript levels after somatostatin administration. Somatostatin suppressed GH-releasing hormone-induced GH promoter activity in a pertussis toxin sensitive manner. Introducing SSTR2 into human somatotropinomas and prolactinomas in primary cell cultures suppressed GH and PRL transcripts, demonstrating that somatostatin suppresses GH transcription at least in part through SSTR2. Interestingly, somatostatin was shown to stimulate GH secretion at low doses (below 10−13 M), an effect that was mediated by SSTR5. Regarding PRL, SSTR5 agonists suppress its secretion, but not its transcription in vitro. Somatostatin analogs suppress pro-opiomelanocortin (POMC) promoter activity, an effect that is abolished following SSTR2 knockdown.
Over the past two decades, somatostatin analogs have become the mainstay of treatment for patients with acromegaly and NET-associated carcinoid syndrome. Further research has increased the understanding of specific SSTR expression within tumors and added to our understanding of the mechanism of action of somatostatin analogs. This knowledge is vital in order to elucidate why somatostatin analogs are effective in the treatment of certain tumor types, but ineffective in others. In addition, these studies have also helped in the development of multireceptor-targeted somatostatin analogs like pasireotide, which has the potential to be effective in a broader range of tumors where SSTRs other than SSTR2 predominate.
Structure and Function of Somatostatin
Somatostatin analogs, such as octreotide, lanreotide, and pasireotide, which function as somatostatin receptor ligands (SRLs), are the main drugs used for the treatment of acromegaly. These ligands are also used as important molecules for radiation therapy and imaging of neuroendocrine tumors. Somatostatin receptors (SSTRs) are canonical G protein-coupled proteins that play a role in metabolism, growth, and pathological conditions such as hormone disorders, neurological diseases, and cancers. Cryogenic electron microscopy combined with the protein structure prediction platform AlphaFold has been used to determine the 3-dimensional structures of many proteins.
Recently, several groups published a series of papers illustrating the 3-dimensional structure of SSTR2, including that of the inactive/activated SSTR2-G protein complex bound to different ligands. The results revealed the residues that contribute to the ligand binding pocket and demonstrated that Trp8-Lys9 (the W-K motif) in somatostatin analogs is the key motif in stabilizing the bottom part of the binding pocket. In this review, we discuss the recent findings related to the structural analysis of SSTRs and SRLs, the relationships between the structural data and clinical findings, and the future development of novel structure-based therapies.[3]
References
[1]Pittaluga A, Roggeri A, Vallarino G, Olivero G. Somatostatin, a Presynaptic Modulator of Glutamatergic Signal in the Central Nervous System. Int J Mol Sci. 2021 May 30;22(11):5864.
[2]Theodoropoulou M, Stalla GK. Somatostatin receptors: from signaling to clinical practice. Front Neuroendocrinol. 2013 Aug;34(3):228-52.
[3]Zhang B, Xue L, Wu ZB. Structure and Function of Somatostatin and Its Receptors in Endocrinology. Endocr Rev. 2025 Jan 10;46(1):26-42.
See also
Lastest Price from Somatostatin manufacturers
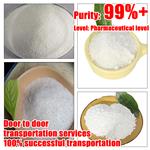
US $1.00/g2025-04-16
- CAS:
- 51110-01-1
- Min. Order:
- 1g
- Purity:
- 99%
- Supply Ability:
- 100kg
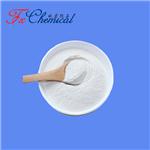
US $0.00/g2025-04-16
- CAS:
- 51110-01-1
- Min. Order:
- 1g
- Purity:
- 98%min
- Supply Ability:
- 1000g